Humans are the only living species of hominin, a tribe of great apes that also includes our shorter, stockier, stronger – and extinct – cousins, the Neanderthals. These prehistoric relatives originated in Europe, colonised Asia and were successful for almost 250,000 years. But within 10,000 years of ‘anatomically modern humans’ appearing in Eurasia, following our last migration out of Africa over 50,000 years ago, Neanderthals had disappeared.
While the cause of extinction remains controversial, many academics believe our ancestors outcompeted Neanderthals by being smarter. Archaeological evidence tells us that we had burial rituals, cave art and tools that surpassed anything created by the Neanderthals – all thanks to an ability to innovate. “We could solve novel problems better,” says Prof Fred Coolidge, a psychologist at the University of Colorado, Colorado Springs, and co-author of How To Think Like A Neandertal.
Unfortunately, we can’t go back in time to meet the Neanderthals and find out what made them tick. But new lab techniques are allowing us to do the previously unimaginable: recreate the Neanderthal mind in the lab. And it could give us the best chance yet of finding out why Homo sapiens survived while Homo neanderthalensis died out.
The social ape
The leading theory for why natural selection has favoured intelligence is the ‘social brain’ hypothesis: being intelligent is an adaptation for dealing with the complex interactions associated with living in groups. Humans manage 150-200 people, for example, whereas average group size in chimpanzees is 50. That figure can double, but tensions soon cause the chimpanzee troop to split. “Neanderthals couldn’t handle groups of more than 20-30,” says Coolidge. More relationships require more brain power and, in mammals, the size of a social group is correlated with the size of the cerebral cortex – the folded, outer layers of the brain, which are involved in higher thought processes such as language and decision making.
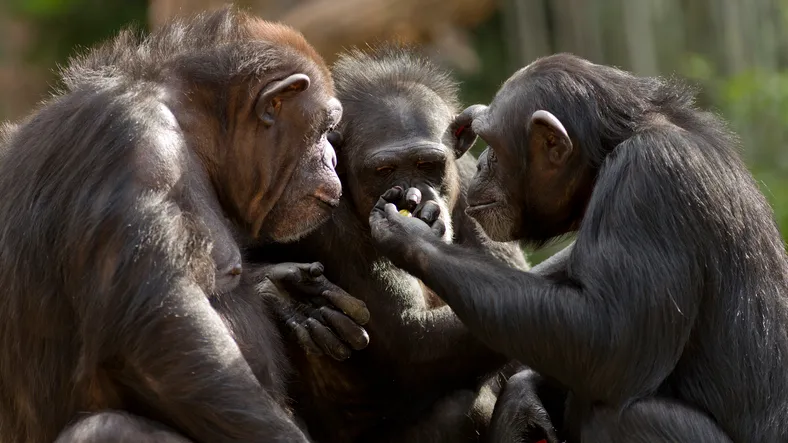
Larger groups enabled ideas to spread. “We are able to collaborate, to cooperate, and to share technology in a way no other species has done,” says Dr Alysson Muotri at the University of California, San Diego. “Part of that is due to the ability to communicate and interact among ourselves – and most of that seems to have evolved from the front of the cortex.”
To find out more about the brains of modern humans and Neanderthals, Muotri is growing organ-like structures made from cells from the frontal cortex. Could these organoids, or ‘mini-brains’, help explain what makes humans unique?
New genetics, old DNA
Growing organoids based on an extinct species is possible due to breakthroughs in several techniques: generating stem cells, extracting DNA from fossilised bones, and gene editing. Muotri is making organoids through a process that he calls ‘Neanderthalisation’. Here, he edits genes in human cells, replacing one letter of DNA with another – the genetic variant carried by Neanderthals – then prompts those cells to develop into pea-sized balls of cortex tissue. The Neanderthal variant is identified by comparing human DNA to the Neanderthal genome, which was first sequenced in 2010 by a team led by Prof Svante Pääbo of the Max Planck Institute for Evolutionary Anthropology in Leipzig, Germany. Incidentally, Pääbo’s lab is also creating Neanderthalised organoids.
One of the first human genes Muotri edited is NOVA1, a ‘master regulator’ which encodes a protein that controls when other genes are switched on or off. NOVA1 is known to be involved in early brain development because its mutations are linked to autism and schizophrenia. As organoids with the Neanderthal variant of NOVA1 mature, they develop defects in the synaptic connections between cells, scrambling their neural networks. Neanderthalised cells within the ball also migrate in a manner that ends up producing unexpected extra folding, says Muotri. “They look like popcorn.”
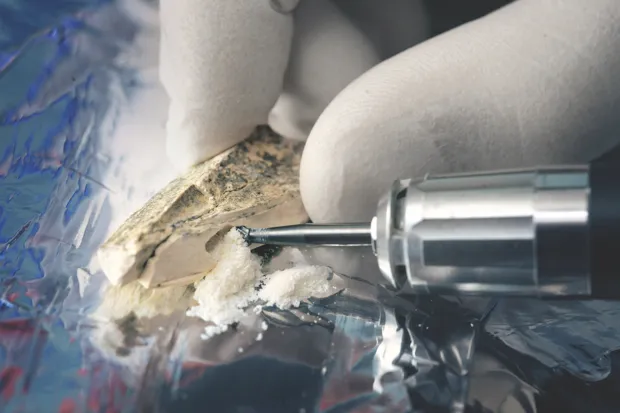
But some scientists are sceptical that organoids will reveal much about our extinct cousins. “Human-type behaviour, and I include Neanderthals, is affected by much more than just the cortex,” says Coolidge, who points out that many daily activities, like walking and talking, are associated with ‘procedural memory’. This involves unconscious processes occurring in regions below the cortex, like the cerebellum, which is responsible for coordinating movement and is also linked to autism.
Acknowledging the limitations of studying the cortex alone, Muotri is hoping to grow a true mini-brain, or ‘cerebral organoid’. “Maybe in the future we will learn how to make all the brain regions and put them together,” he says. Editing a single gene – NOVA1 – technically produces ‘partially Neanderthalised’ cells, so he’s now working to make a ‘fully Neanderthalised’ organoid, which requires replacing DNA letters in thousands of genes. “We are replacing chunks of the entire chromosome in these cells.”
The best model to study the human brain is in a living person, but society considers such experiments unethical, so scientists use surrogates – anything from a few cells in a Petri dish to grafting tissue onto a mouse brain. So where do mini-brains lie on the ethical scale? According to Prof Hank Greely, a bioethicist at Stanford Law School, they’re currently too small and simple to cause concern. “If organoids become larger and more complicated, then I think you’re a lot closer to having to worry about what’s in the dish.”
Regardless of which surrogate is used as the model for a living brain – human or Neanderthal – Greely says the key ethical question is the same: would it be entitled to special treatment? The answer isn’t based on whether it deserves ‘human’ rights, but on considerations that already apply to laboratory animals, like the capacity to feel pain (as detected through characteristic patterns of neural activity). Such barriers would probably prevent scientists from creating mini-brains capable of consciousness or becoming sentient.

But even if technical hurdles can be overcome and the research is passed by an ethical review panel, growing an entire Neanderthal brain may not offer enough insight into the lives of our extinct relatives, as it would be difficult to understand the behavioural impacts of any Neanderthal-human DNA differences in a brain that’s isolated from its environment. So if the aim is to understand the Neanderthal mind, why not grow a whole body too, by cloning?
Cloning a Neanderthal has been suggested by renowned geneticist Prof George Church. It would involve extensive gene editing to generate a fully Neanderthalised embryo, implanted into a human mother. Bringing a Neanderthal baby kicking and screaming into the 21st Century means de-extinction of the species, and Greely says the ethical implications run deeper, as he suspects people wouldn’t be civilised enough to treat clones with respect, partly due to racism illustrated by the outdated ‘stupid caveman’ stereotype. “Even if we didn’t have that image, just knowing that they were not fully human would lead to discrimination,” he says.
Mental trade-offs
Views on our extinct relatives have shifted recently. While this shift can be explained by archaeological finds that show a more sophisticated Neanderthal culture than once assumed, the cynical explanation is that the shift has only happened since present-day Europeans discovered that interbreeding occurred between the two species. Today, non-Africans carry about 2 per cent Neanderthal DNA.
The legacy of mating can still be heard in our anatomy. “Even the small echoes of Neanderthal DNA that we all harbour are very much active in modern humans,” says Dr Karen Berman, chief of the neuroimaging section at the US National Institute of Mental Health. In 2017, Berman and her colleague Dr Michael Gregory performed MRI scans on 221 healthy humans to construct 3D models of their heads. The work revealed that people with a greater number of ancient genetic variants – a higher ‘NeanderScore’ – have skull shapes that more closely match our extinct cousins, whose skulls were elongated at the back. (Although we associate size with smarts, the Neanderthal brain was actually 10 per cent larger.)
After sequencing DNA from the participants’ blood samples, Berman and Gregory found that a higher NeanderScore also meant the cortex had more grey matter (brain cells) and white matter (mostly branching fibres from cells). Folding of the cortex was greater too, mirroring Muotri’s popcorn-like organoids. The most affected areas were beneath the back of the skull: the occipital and parietal lobes of the cortex – regions that are involved in processing visual and spatial information.
The brain has limited resources, so allocating more to visual and spatial processing comes at the expense of other abilities, leading to a trade-off. Those genetic variants that influence how a brain’s resources are allocated seem to have given Neanderthals superior visual and spatial skills (probably for hunting), driven by ecological pressures to find calorific food to fuel an energy-hungry body in a cold climate. With modern humans, on the other hand, the trade-off meant that we had more processing power for social skills, contributing to our evolutionary success.

Ancient variants continue to affect humans today, as revealed by certain mental illness and neurological disorders. For example, ‘neurotypical’ people have one copy of a set of 25 genes located on chromosome 7, whereas a person with the condition ‘Dup 7’ has a duplication that creates two copies of each gene. This provides better visual and spatial skills but makes them less sociable – as in autism. At the other end of the spectrum, ‘Williams syndrome’ results from a deletion of those same 25 genes, leading to poor visual and spatial skills but hypersociability, which produces someone with a ‘gregarious brain’ who has a compulsion to strike up conversations with strangers.
So studying the impact of genetic mutations by comparing organoids, including those with ancient variants found in Neanderthals, can provide insights into conditions that affect modern humans. “This could provide information not only about what makes us who and what we are, but also possibly pave the way for treatment and diagnostic approaches,” explains Berman.
That’s actually the main reason why Muotri is growing mini-brains. His lab contains thousands of mutant organoids for various diseases. He’s already found that, like Neanderthalised structures, ‘autistic’ organoids have defects in their neural networks. “One misconception many people have is that by studying the evolution of the brain, there is no benefit to human health,” says Muotri, whose son suffers from autism. “By understanding how the social brain evolved, we might be able to create better therapeutics.”
“Many academics believe our ancestors outcompeted Neanderthals by being smarter”
This is an extract from issue 328 of BBC Focus magazine.
Subscribe and get the full article delivered to your door, or download the BBC Focus app to read it on your smartphone or tablet. Find out more
Why are mini-brains being used to control robots?
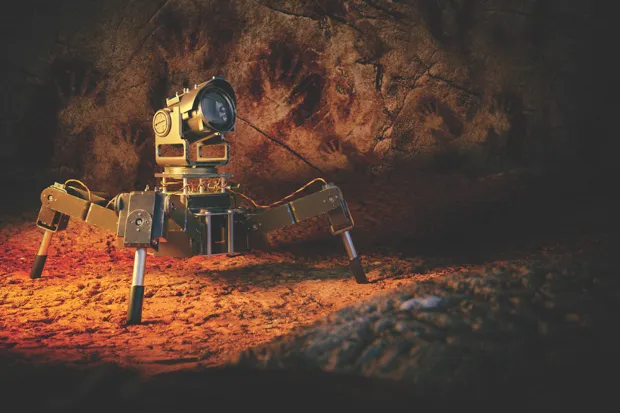
Neuroscientist Alysson Muotri is putting organoids inside four-legged, spider-like robots. The main reason for such an unusual mind-machine interface is that it enables the ‘brains’ to explore the world around them – via an artificial body. “They’re similar to a newborn baby who’s trying to get used to touching things and experiencing different sensations,” Muotri explains.
Electrical activity rises as an embryonic brain develops but reaches a plateau before birth, needing external input from the senses for its neural circuits to mature. In a spider bot, electrodes capture activity from the organoid’s cells and split the electrical output between the robot’s limbs, which must then synchronise. “In a very simplistic way, that’s exactly what the human brain does when a baby is trying to crawl or walk,” says Muotri. “So we’re teaching the organoid how to make this spider robot walk, by coordinating movements of the legs.”
It’s already learning. The ‘brain’ can currently tell its body to move forward; the next step is teaching it to go backward when it encounters an obstacle, which requires providing feedback for when a movement is wrong. That brain training is being achieved in two ways. The first method is a form of ‘mind control’ called optogenetics, in which cells are genetically engineered to to be sensitive to light so that their behaviour can be controlled. The second method involves supplying cells with a dose of the reward hormone, dopamine. Both methods cause the organoid’s neural networks to rearrange, allowing it to adapt.
Once organoids are able to learn, they can battle in robot wars – obviously not a fight, but a competition of speed. An organoid made from Neanderthalised cells with a NOVA1 gene variant has defects in synaptic connections between its cells, affecting its neural networks. Would that make it slower to adapt compared to a human organoid? While speculative, the results could offer clues on whether our human ancestors were faster at thinking, influencing their behaviour.
The spider bot, with its multiple legs, is allowing Muotri’s lab to observe how a single organoid handles coordination. One follow-up experiment would be to see how several organoids deal with cooperation. Would they interact to accomplish a common goal? The design for cooperating robots is still in the planning stages – it might involve two or three human-like arms that must work together to lift an object, or robotic versions of social insects like ants and bees. “This might sound like science fiction,” says Muotri, “but it is actually happening, and they have a clear purpose.”
Follow Science Focus onTwitter,Facebook, Instagramand Flipboard