The year is 1869 and a young researcher is toiling away in a laboratory in an old castle in Germany, on course to make a remarkable discovery. The lab studies the composition of cells, and Friedrich Miescher is analysing relatively simple white blood cells, which he extracts from the pus in a local clinic’s discarded bandages.
Having exhausted his efforts in classifying the cell’s proteins, Miescher turns his attention to another substance that keeps appearing in his samples. He finds it odd – an acid that contains phosphorus – and declares he has discovered a completely new type of substance. Nuclein, or DNA as we now call it, has been found.
Like any good sceptical scientist, Miescher’s boss Felix Hoppe-Seyler is wary and waits to repeat the experiments before, two years later, allowing publication. But this delay would turn out to be negligible; it was many more decades before scientists saw the importance of DNA.
Misecher went on to find DNA in a variety of cells, but even he couldn’t believe that just one substance generated the enormous diversity of life. The race to understand the discovery of DNA was on.
As late as the 1940s, most scientists thought that proteins – large biological molecules which come in all shapes and sizes – were the only substances complex enough to be the agents of heredity.
Read more about DNA:
- Instant Genius: DNA
- The origin of life
- A genetic mutation could help us understand how to stop pain
- Growing up poor can affect your DNA as well as your health
Chromosomes, the coils of DNA and protein that contain genes, had first been spotted in cells in the early 1840s. Later that century, researchers saw them double in number and then halve again into separate ‘daughter’ cells during cell division. In 1865, the Austrian monk Gregor Mendel used pea plants to explore theories on genetic inheritance, proposing that characteristics are inherited in discrete units.
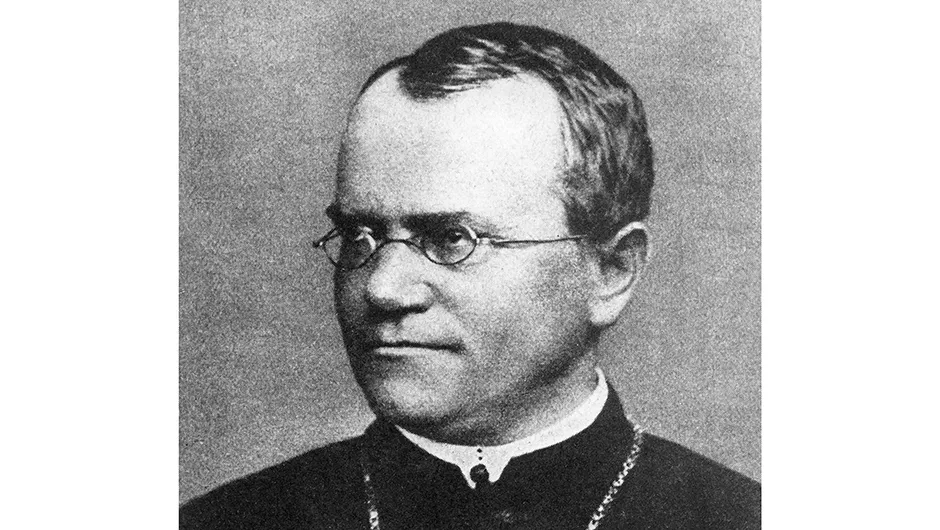
When his research was rediscovered in the early 1900s, a flurry of work determined that these units, or genes, must be in chromosomes. But what were they made of – DNA or protein – and what did they look like?
A German doctor named Albrecht Kossel made some of the first steps towards finding out. Working under Hoppe-Seyler in the late 1800s, he discovered DNA’s ‘bases’ (the chemical opposite of acids) and named them thymine (T), adenine (A), cytosine (C) and guanine (G).
This work was continued by Phoebus Levene, a Lithuanian researcher driven to New York in the early 1890s because of anti-Semitism in his adopted home of St Petersburg. For three decades from the mid-1890s, Levene studied the structure of DNA, identifying its other components: a sugar called deoxyribose and phosphate groups.

He also discovered that DNA is made up of units that he called nucleotides. Each of these is made up of a sugar, phosphate group and base, and they are linked by bonds between the phosphate groups of one nucleotide and the sugar of the next, forming a so-called backbone.
But this was as far as his correct findings went. He thought that each DNA molecule contained only four nucleotides, one with each type of base, linked together in a ringhe called a ‘tetranucleotide’.
Levene’s tetranucleotides were too simple to carry a genetic code, and so reinforced the idea that proteins must be the hereditary agent. Revealing DNA’s hidden complexity was going to require a closer look. While Levene was unravelling the complexities of DNA in New York, across the Atlantic a father-and-son team was establishing a technique that would prove key to determining DNA’s structure.
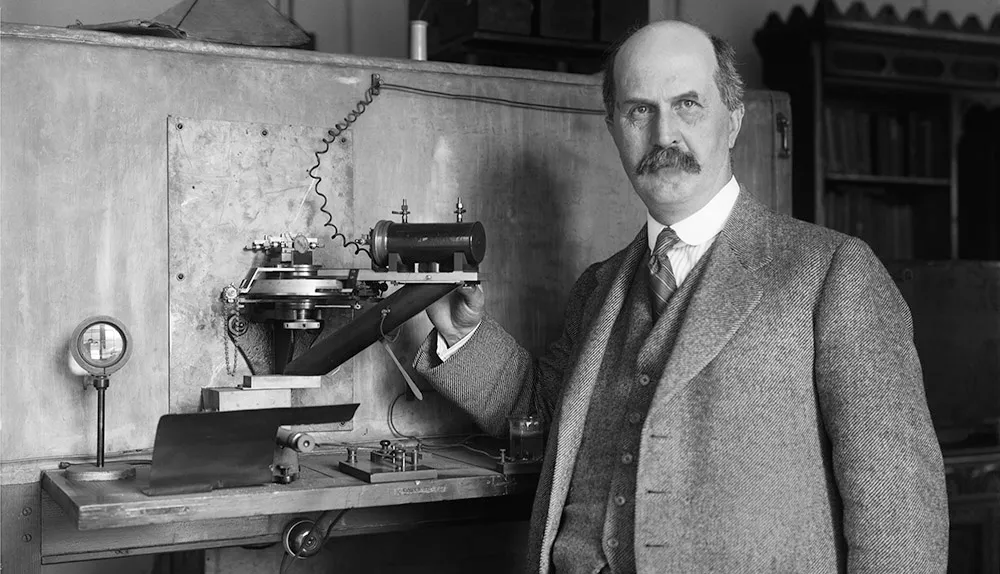
William Henry Bragg, a physicist at the University of Leeds, and his son William Lawrence Bragg, a researcher at the Cavendish Laboratory in Cambridge, laid the foundations for the field of X-ray crystallography between 1912 and 1914.
They were inspired by the work of Max von Laue, who discovered in 1912 that X-rays bend when they pass through crystals, substances with highly ordered structures. The younger Bragg reasoned that because they have ordered patterns of atoms, the way the X-rays bend through crystals would reveal something about their structure.
His more practically minded father built the first X-ray spectrometer – a device for shooting a narrow beam of X-rays at substances – and together they tested the theory on salt crystals.
In these experiments, they placed a photographic plate behind the crystal, onto which the scattered X-rays would produce a characteristic pattern. William Lawrence Bragg came up with an equation, known as Bragg’s Law, which allowed them to work backwards from the patterns to deduce the crystal’s structure. The pair won a Nobel Prize in 1915.
Key terms
Nucleotide
The basic subunit of DNA. Each nucleotide is made up of a base (the ‘letters’ of DNA: adenine, guanine, thymine or cytosine), a sugar and a phosphate group. The nucleotides form the two parallel complementary chains of DNA, with adenine matched to thymine and guanine to cytosine.
Phosphate group
A phosphorous atom surrounded by oxygen atoms. Phosphate groups, along with deoxyribose sugars, make up the ‘backbone’ of the long DNA molecule.
X-ray crystallography
This is the study of the structure of crystals by firing X-rays at them. The X-rays bounce off the regular arrangements of atoms in crystals, and the patterns they make are captured on film. An equation is then used to work out the crystal’s structure.
One of the first groups to apply this technique to biological molecules was headed by William Astbury, who began working at the University of Leeds in 1928, having studied under William Henry Bragg at the Royal Institution. In 1937, Astbury was sent samples of calf DNA by Swedish researcher Torbjörn Caspersson.
A few years previously, Caspersson had shown that DNA is a polymer – a long chain of nucleotides – rather than the short lengths Levene had suggested. Astbury’s PhD student, Florence Bell, took the first of hundreds of X-ray diffraction pictures of DNA that year.
The fact that it produced a pattern at all suggested that DNA had a ‘solvable’ structure. Astbury and Bell’s pictures look like smears compared to the clear images that Rosalind Franklin produced in the early 1950s, but they did reveal one crucial fact: the distance between the bases in the DNA molecule.
In 1938, Astbury used the images to propose a structure for DNA in which the bases are stacked on top of each other, but the pictures weren’t detailed enough for him to get much further.
The race to find the structure of DNA
Meanwhile, back in the US, a medical researcher named Oswald Avery was busy refining a 1928 experiment by a British microbiologist called Fred Griffith. He had shown that it was possible to make harmless bacteria and their progeny dangerous by mixing them with virulent bacteria, suggesting that something was being transferred from the virulent to harmless bacteria.
Avery and his colleagues deliberately created conditions in which only DNA – not protein – could be transferred.
In this way, they determined that only DNA could pass on traits. Though many would refuse to believe it, DNA had been strongly implicated as the carrier of inheritance, and science had the tools to find out what it looked like. The stage was set for the race to find the structure of DNA in the 1950s – only not everyone knew it was a race.
Read more about the discovery of DNA:
- Photo 51: the key discovery behind the structure of DNA
- DNA: a timeline of discoveries
- Understanding DNA: Five key scientists who unravelled the helix
- Who really discovered DNA?
DNA research was to benefit from the post-WWII mood in science, as many physicists who had been employed in war work turned their attention to the more benign biological problems. Among them was Maurice Wilkins, who had worked on both radar and the Manhattan Project to build an atomic bomb.
By the middle of 1950, Wilkins was assistant director of King’s College London’s new biophysics unit. In a dank basement underneath the Thames, Wilkins and PhD student Raymond Gosling were producing much sharper X-ray pictures of DNA than Astbury had managed.
Rosalind Franklin was invited to join the unit’s DNA research in 1951, bringing with her important crystallography skills after making her name in Paris with X-ray insights into the structures of coal, carbon and graphite. But misunderstandings with Wilkins over her role in the DNA research caused a rift that arguably cost them the scientific race.
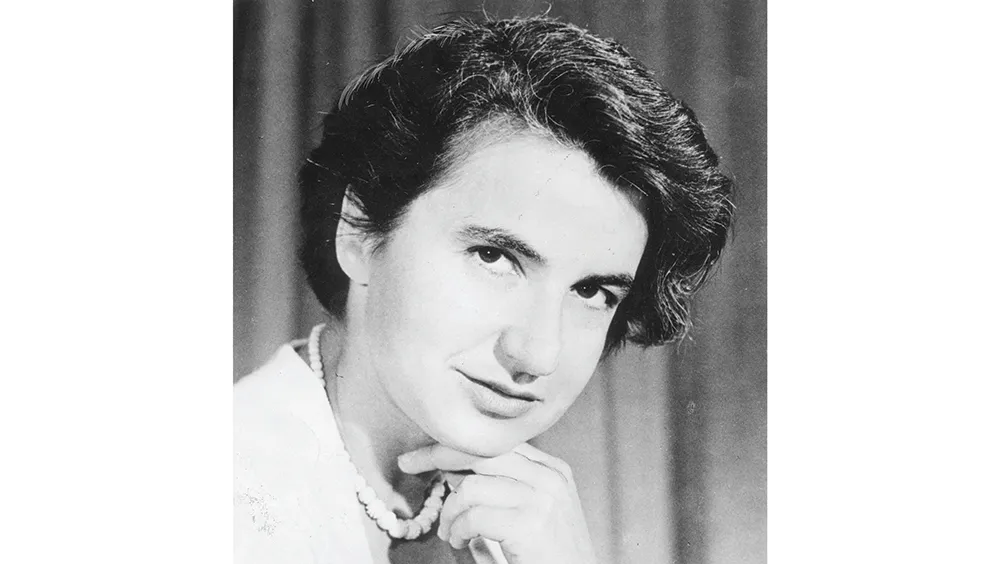
One of the biggest discoveries Franklin made in her time at King’s was to discover, along with Gosling, that there are two forms of DNA: a dehydrated, tightly packed ‘A’ form and a hydrated, longer ‘B’ form, which produced different X-ray patterns. Astbury’s blurry images must have been a combination of the two.
The King’s group, and Franklin in particular, believed that the structure would emerge from careful X-ray work. But at the Cavendish Laboratory in Cambridge, now headed by William Lawrence Bragg, a pair of researchers called James Watson and Francis Crick had other ideas.
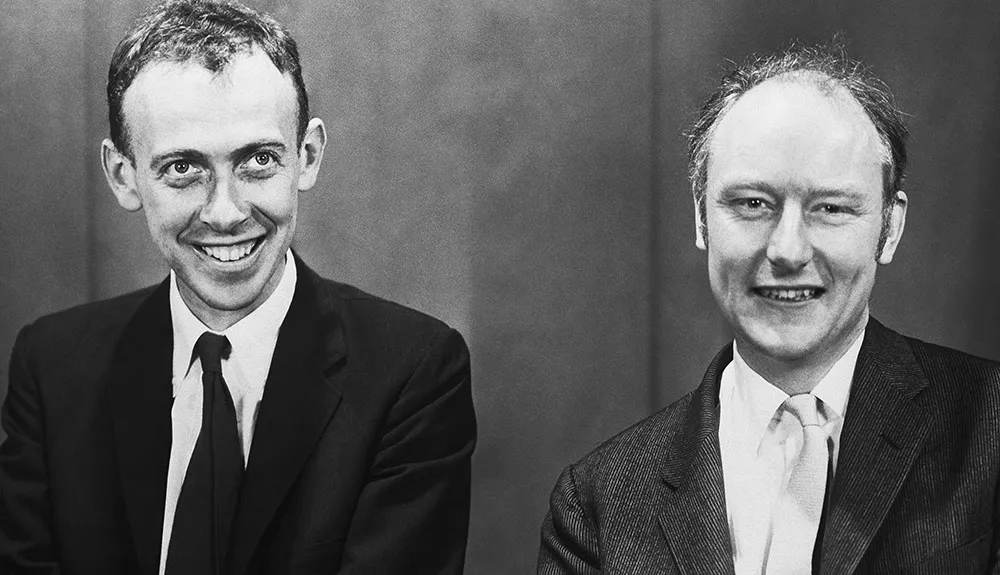
Photo 51: the key to the puzzle
Watson, an American researcher in his 20s who’d gained his PhD at an unusually young age, and Crick, older with a reputation for a sharp mind, did famously little in terms of experiments with DNA. Instead they chose to build physical models to work out how DNA’s known components could fit together. Much of their experimental knowledge came from seminars and informal conversations with Wilkins, with whom they were on friendly terms.
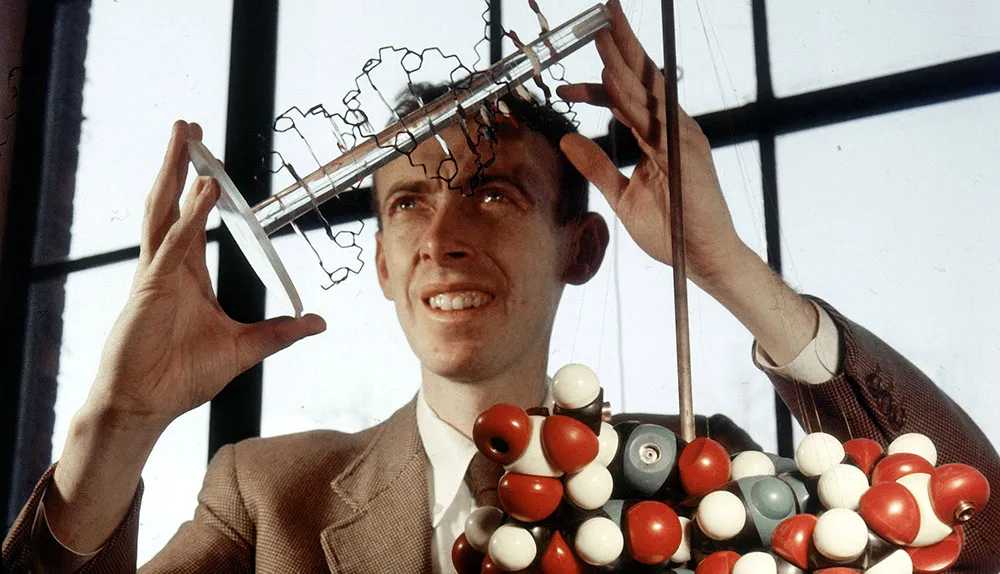
At the end of 1951, Watson and Crick invited the King’s team to see their latest model, which they believed to be the structure. Informed by Watson’s memory of a talk by Franklin, it was made up of three DNA chains with the sugar-phosphate backbone on the inside and the bases on the outside. Franklin immediately knew it was wrong – DNA’s water content meant the backbone had to be on the outside. Embarrassed, Bragg banned the pair from any more DNA work.
In May 1952, Franklin took Photo 51 – a stunningly clear picture of the B form of DNA. Abiding by an earlier agreement with Wilkins to focus on the A form, she put it aside.
By January 1953, Franklin had decided to leave King’s for Birkbeck College and began sharing her work with Wilkins. Wilkins, who had long believed that DNA was a helix, showed the image to Watson, who later wrote: “The instant I saw the picture, my mouth fell open and my pulse began to race.”
Photo 51 immediately spelt out ‘helix’ to Watson, and he returned to Cambridge inspired.

In February 1953, Linus Pauling, a giant of molecular biology with expertise in protein structure, proposed his own structure. But with only Astbury’s earlier data to go on, he got it wrong. Among other basic mistakes, he suggested that DNA was comprised of three chains.
Watson and Crick, concerned that Britain would lose the race, and seeing a chance for themselves, returned to their model-building. They knew how far apart the bases were, that DNA’s backbone was on the outside of the molecule, that the overall structure was a helix, and that it was probably made of two chains.
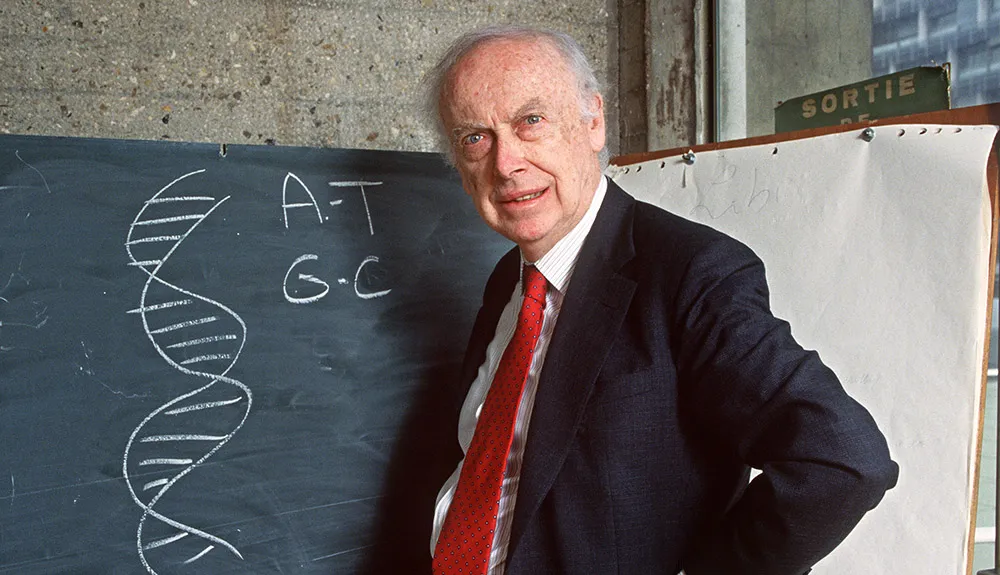
They also saw more of Franklin’s data, this time via a report to the biophysics committee of the Medical Research Council, which funded both groups. From this, Crick was able to deduce that the chains in the DNA molecule look the same upside-down, and must therefore run in opposite directions.
The final piece of the puzzle was a 1949 experiment by Erwin Chargaff, who had visited the Cavendish team in 1952. He determined that the number of As matched the number of Ts, and that the number of Cs matched the Gs. Watson and Crick realised that As must always bond to Ts, and Cs to Gs, producing a ladder-like helix with the paired bases forming the rungs and the sugar-phosphate backbones the sides.

Model completed, the pair went for lunch in a nearby pub called The Eagle and declared that they had found the meaning of life. When the King’s team visited this time, they accepted the model immediately. “Rosy’s instant acceptance of our model at first amazed me,” Watson wrote later. “Nonetheless… she accepted the fact that the structure was too pretty not to be true.”
Crick and Watson’s structure was published in the journal Nature in April 1953, along with two articles from King’s. None revealed the role that King’s data had played, and Franklin died in 1958, perhaps never having known. Watson, Crick and Wilkins shared the Nobel Prize in 1962.
With no-doubt knowing understatement, Watson and Crick wrote in their 1953 paper: ‘It has not escaped our notice that the specific pairing we have postulated immediately suggests a possible copying mechanism for the genetic material.’
In the years since 1953, researchers have learned how DNA copies itself and how its strings of As, Ts, Cs and Gs provides a template for making proteins. More recently, analysis of the human genome has allowed scientists to glimpse the intricacies of how DNA orchestrates life.