In 2016, Wieger Wamelink, a plant ecologist based at Wageningen University, sat down at the New World Hotel in the Netherlands with 50 guests for a one-of-a-kind meal.
Things might have looked ordinary enough from a quick glance at the menu, if maybe a little cheffy – pea puree appetisers to start, followed by potato and nettle soup with rye bread and radish foam, then carrot sorbet to finish.
But the thing that made it such an extraordinary occasion was that all the vegetables used to make the meal had been grown in simulation Martian and lunar soils by Wamelink and his team.
Since then, they have grown an impressive 10 crops, including quinoa, cress, rocket and tomatoes using simulation soils produced using crushed volcanic rocks collected here on Earth. The team produced their simulant soil by grading the particles of rock into different sizes and mixing them in proportions that match rover analyses of the Martian soil.
Read more about living off Earth:
- NASA's Martian habitat contest final stage winners announced
- Move over, Mars: why we should look further afield for future human colonies
- The thought experiment: What would living on Mars do to my body?
The soils were initially developed so that rovers and spacesuits could be tested on Earth to see how well they handled the surface materials of Mars and the Moon. Few thought that the soils could ever actually be farmed.
For a start, there were concerns about the texture of the soil, especially after early attempts to farm model lunar soils struggled as a result of tiny, razor-sharp rock fragments that punctured the plants’ roots. On Mars, though, the movements of ancient water and ongoing wind erosion have left a far more forgiving surface covering on the planet, and the simulation soils have proved to be successful.
Nutritionally, Wamelink says there’s no difference between the ‘Martian’ crops and those grown in local soils, and when it comes to flavour he was most impressed by the tomatoes’ sweetness.
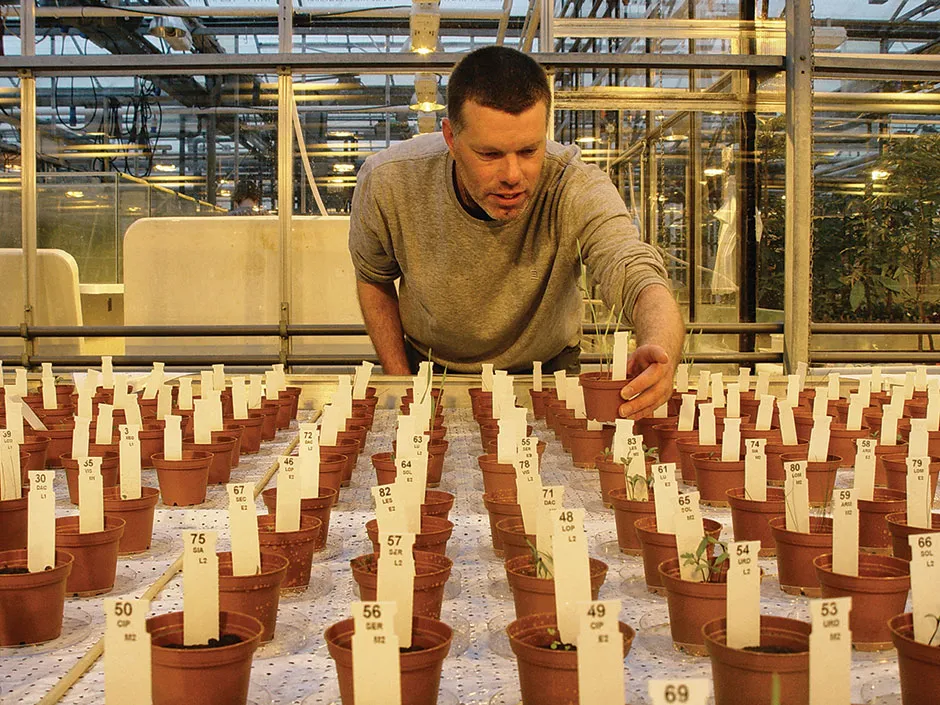
Wamelink and his team are now attempting to improve crop yields by infusing the simulation Mars soil with nitrogen-rich human urine, a resource likely to be readily available on crewed missions to the Red Planet. He also plans to introduce bacteria that will fix more atmospheric nitrogen, and also feed on the toxic perchlorate salts present in Mars soil.
Elsewhere, at Villanova University in Pennsylvania, Prof Ed Guinan and Alicia Eglin are leading the Red Thumbs project, and have had several successes in farming their own Martian simulant.
Initially derived from rocks gathered in the Mojave Desert, the Villanova researchers have augmented their model soil with earthworm farms, due to the animals’ ability to release nitrogen from dead organic matter through their burrowing and feeding.
The Red Thumbs project made headlines in 2018 when the international media got excited about the prospect of Martian beer, after Guinan and Eglin’s team managed to successfully produce barley and hops.
All the salad you want, but no chips
A couple of years on and Guinan and Eglin have now added tomatoes, garlic, spinach, basil, kale, lettuce, rocket, onion and radishes to their greenhouses. The quality of harvests has varied, but chief among the successes was kale, which actually grew better in the simulant Martian soil than in local soils.
Other crops struggled, such as the much-needed and calorie-dense potatoes. It turns out potatoes prefer more of a loose, uncompacted soil and failed to grow as the simulant soils became heavy and impenetrable when watered, which led to the potatoes being choked out.
Eglin believes that the key to success may be to grow lower yield crops that might enjoy more natural ecosystems than a single-species setup would allow. Even on Earth, agricultural monocultures often suffer over time as nutrients essential for that one plant being grown are progressively depleted and not replaced after each harvest.
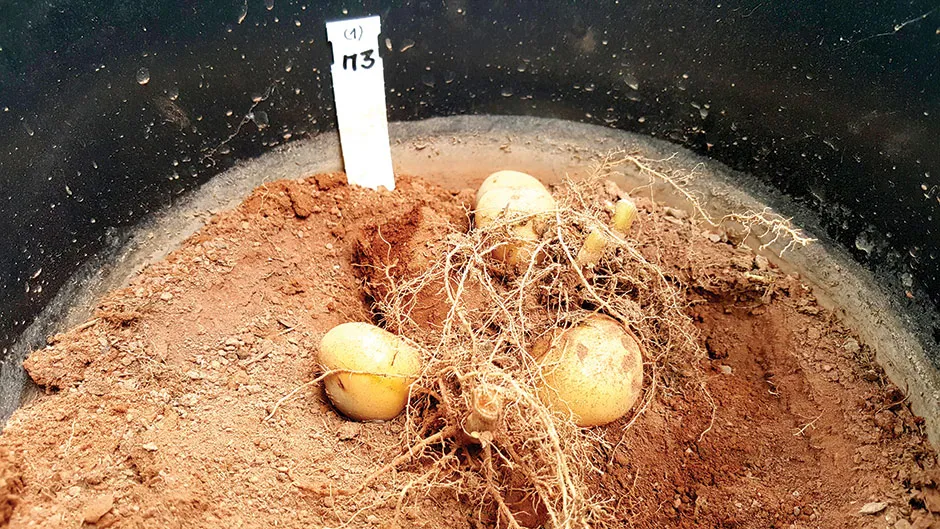
To counteract this effect, farmers often introduce secondary species in the same growing area. These wouldn’t compete with the main crop, because their root systems are shallower, but they would still offer additional nitrogen fixation to improve soil fertility. Eglin is now planning to test this by growing soybeans, which could prove to be a vital source of protein, and corn alongside pigweed, a leafy vegetable famous for its use in the Caribbean stew callaloo.
But however much success these projects have, we must remember that simulant soils have very real limitations, explains ESA’s Christel Paille. She’s involved in the Micro-Ecological Life Support System Alternative programme (MELiSSA), which is exploring a range of technologies for use in long-haul, crewed missions, such as bacterial bioreactors that recycle astronaut waste into air, water and food.
While MELiSSA has provided support to Wamelink, Paille points out that any successes from the model soils must take into account the fact that they’re based on limited geographical sampling.
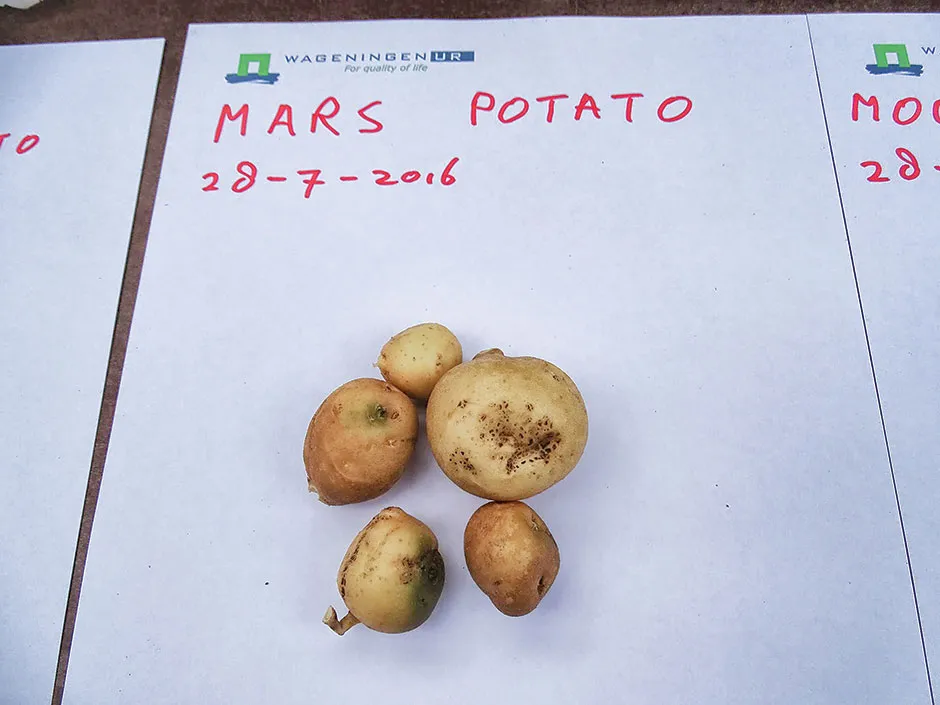
“It’s a baseline, but probably not something that we can generalise to any location on the Mars surface. We are always very cautious about a simulant material. It’s very difficult in a single simulant to capture all the characteristics [of the Martian surface],” she says.
Perhaps the only way around this is to collect a sample from the surface of Mars and return it to Earth. On 30 July, NASA’s Perseverance Rover launched from Cape Canaveral in Florida with its sights set on the ancient river delta deposits in Mars’s Jezero Crater. If all goes according to plan, next February the rover will find itself in what’s thought to be some of most fertile land on the Red Planet.

Thanks to its plutonium-based power system, the rover should be able to spend up to a decade analysing the surface of Mars. While previous missions have looked for signs of habitable conditions that existed in the past, Perseverance aims to go one step further by searching for signs of past microbial life.
Also, and crucially for those with hopes of growing food on Mars, the rover will collect samples of rocks and soil, and store them in preparation for a potential future robotic mission to return them to Earth for analysis. Until then, the simulation soils are all we have to work with.
There is still much to be learned in the meantime. For instance, rather than commit to individual species, Paille’s MELiSSA programme prefers to assess plants within a self-contained, life-supporting ecosystem.
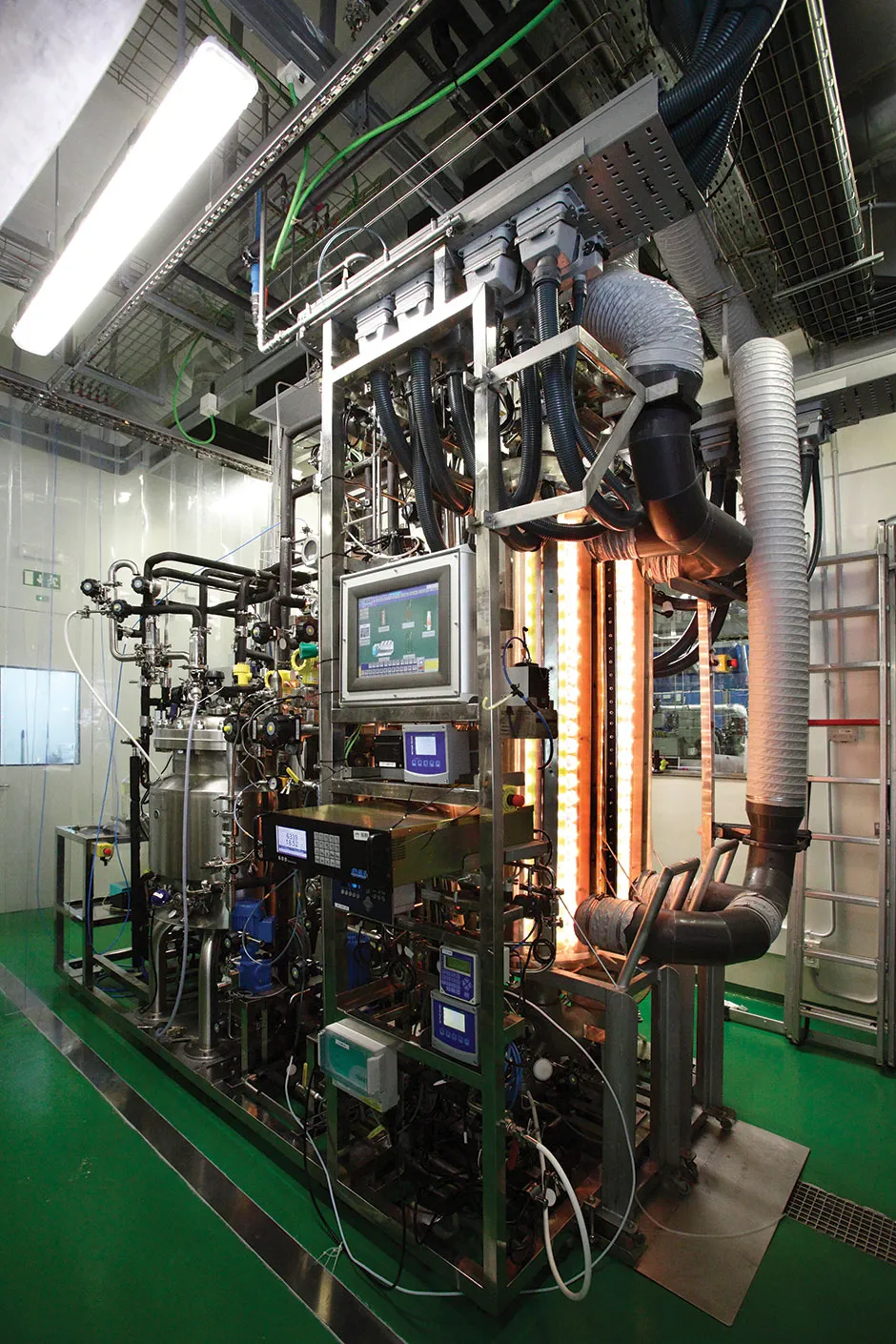
Here, the benefits of edible biomass, oxygen production and even water treatment are balanced against the resources to grow each plant and manage their waste. But predicting crop performance on Mars will require a more fundamental understanding of plant biology.
“It’s about going down to the molecular scale,” says Paille. “We need to characterise what’s happening underground, like in root respiration. How are gases such as oxygen taken up and provided to the root. And how does the carbon dioxide produced actually diffuse out?”
Barriers to growth
Even if a suitable simulant is developed, there are still other challenges to overcome. Mars is located in an orbit that’s around 70 million kilometres further out from the Sun than Earth. As a result, sunlight delivers only 43 per cent as much energy, leaving average temperatures languishing around -60°C. Also because of the planet’s tilt and highly elliptical orbit, seasonal variations are extreme.
Another hurdle is the Martian atmosphere, which is much thinner than Earth’s and lacking in the nitrogen vital for plant growth. Instead it’s dominated by carbon dioxide, which is vital for photosynthesis, but it’s at such low concentrations that any plants growing on the surface would struggle to harness enough to spur growth.
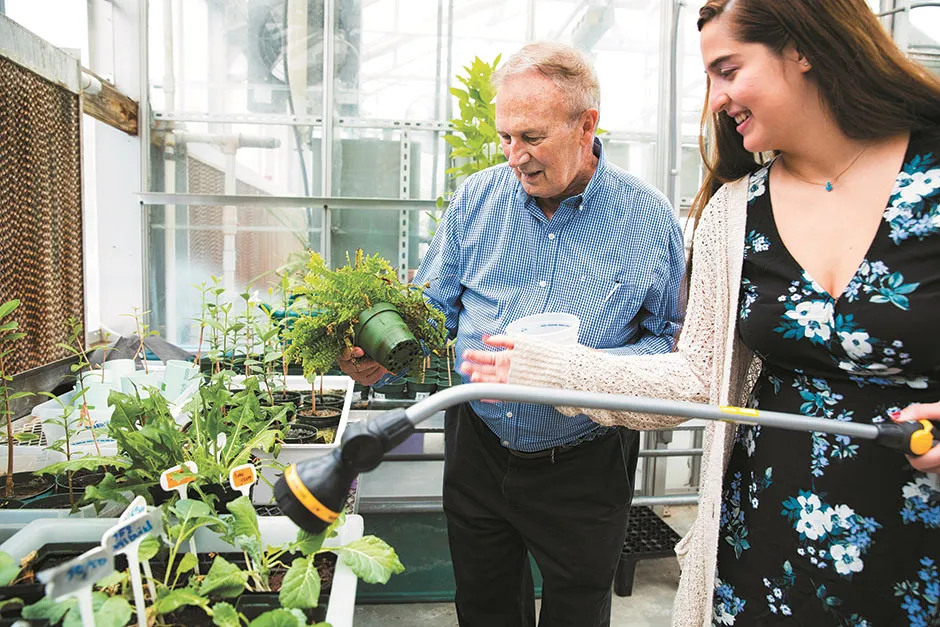
The thin atmosphere also exposes the Martian soil to cosmic radiation. This creates a hostile environment for any microorganisms you might introduce to recycle nutrients from dead plant matter.
Also, Jennifer Wadsworth at the UK Centre for Astrobiology has shown that solar radiation can activate chlorine compounds in Martian soil, turning them into toxic perchlorate salts. These are poisonous if eaten and can lead to hypothyroidism, which blocks the release of metabolism-regulating hormones. Poisonous heavy metals such as cadmium, mercury and iron found in the soil also pose their own challenges.
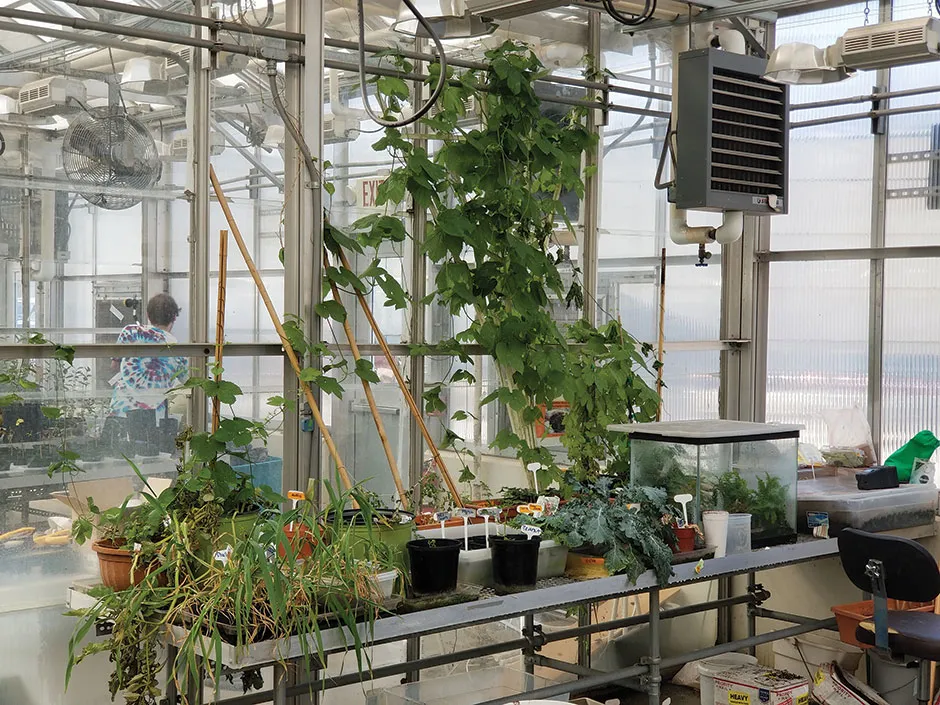
“Everything that’s poisonous for people you can think of in terms of heavy metals is in those soils,” says Wamelink. “For plants it’s not a problem because they’ll store it somewhere. But if we eat those plants then it might be [a problem for us].”
Another option may be soil-less techniques already used on Earth. Aeroponics sees plants suspended in the air while their roots are sprayed with a nutrient mist. Alternatively, hydroponics dips the roots into a nutritious liquid.
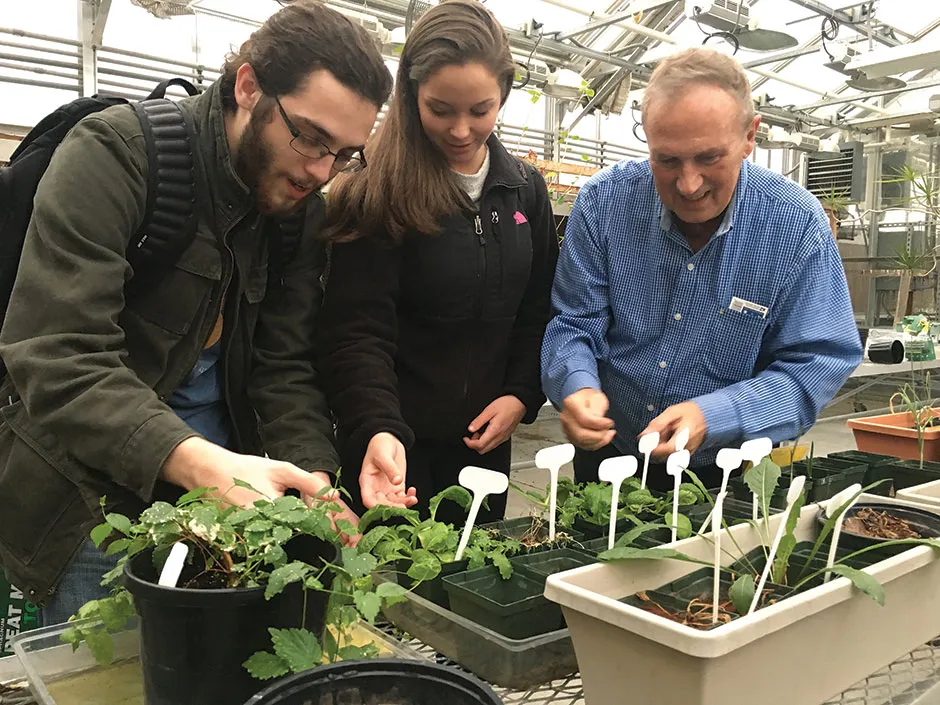
These approaches can produce larger, faster-growing crops, and have already been used to successfully grow lettuce on the International Space Station (ISS). In fact, the astronauts were so pleased with their harvest, says Wamelink, the scientists back home were disappointed with the amount of lettuce samples returned for analysis after too much was eaten.
Calorie deficit
Despite the popularity of the ISS lettuce, air or water agriculture alone may not be enough to sustain astronauts on long-haul trips to Mars, thanks again to the problem of growing potatoes.
“It’s very difficult to grow potatoes in hydroculture, and just eating lettuce and tomatoes won’t be enough because you need calories,” says Wamelink. “Potatoes grow much better in soils, where you’ll get a lot of harvest per cubic metre and the organic matter that you don’t eat can be recycled.”
Whether grown in soil, water or misty air, food will likely play much more than a simple nutritional role in any Martian outpost. Sitting down to a proper meal would prove invaluable for the mental health and comfort of any pioneering astronauts living millions of kilometres from home. Who knows, maybe rye bread and radish foam will be on the menu after all.
- This article first appeared inissue 354ofBBC Science Focus–find out how to subscribe here