How do you detect the undetectable? That’s the challenge scientists have faced since the most ghostly, elusive subatomic particle, the neutrino, was posited nearly a century ago.
Yet now they’ve been detected. In June, neutrinos generated from elsewhere in the Milky Way were spotted. It’s the first time we’ve seen anything but photons of light from our Galaxy. The particles are tiny, but the discovery is huge.
“We’re truly at the dawn of neutrino astronomy,” says Steve Sclafani of the University of Maryland, one of the scientists involved in the project.
It’s fair to say neutrinos are strange. They’re the second-most abundant subatomic particles in the Universe, after photons, but they’re extraordinarily elusive. So rarely are they stopped by anything in their path that, when Austrian physicist Wolfgang Pauli proposed their existence in 1930, he apologised to his fellow physicists.
“I have done a terrible thing,” he said. “I have predicted a particle that can never be detected.”
To get an idea of just how elusive they are, hold up your thumb. Every second, about 100 billion neutrinos from the core of the Sun stream through your thumbnail, and almost none of them are stopped by it.
Pauli bet a case of champagne that nobody would ever detect a neutrino. But towards the end of his life, he lost that wager.
On 14 June 1956, Frederick Reines and Clyde Cowan announced that they’d picked up neutrinos streaming out of a nuclear reactor at the Savannah River Plant in South Carolina. Their trick was to create a target that contained a vast number of atoms, thus boosting the chance that at least one of the elusive neutrinos would be stopped.
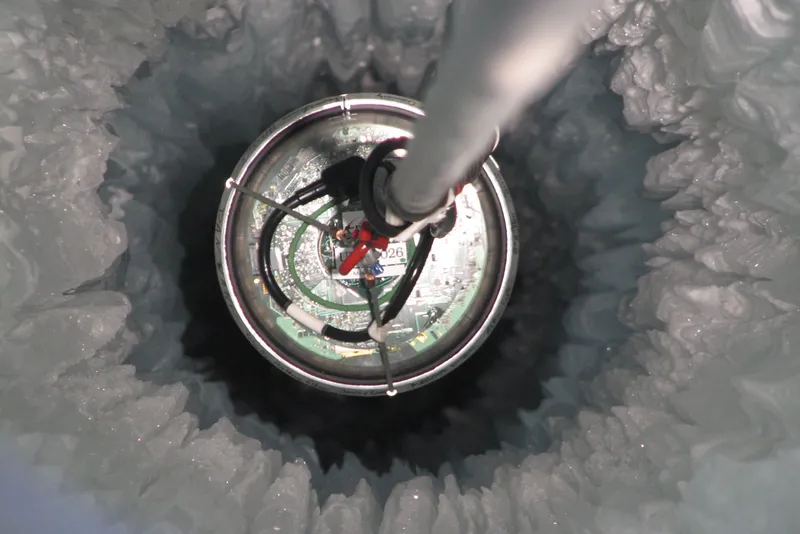
Tiny particles, gigantic target
Creating an unmissable target is the same strategy used by the physicists who recently detected neutrinos from out in the Milky Way. Their target was gigantic: a 1km3 block of ice near the Amundsen-Scott South Pole Station in Antarctica.
The detector, aptly named the IceCube Neutrino Observatory, is operated by a group of around 300 scientists from 58 institutions, led by the University of Wisconsin-Madison. It looks for neutrinos with energies thousands of times greater than anything that can be created in the proton-proton collisions at the Large Hadron Collider near Geneva.
Occasionally, a high-energy neutrino passes through the IceCube detector and shatters an atomic nucleus in a water molecule. This results in a cascade of subatomic shrapnel, with some of the particles travelling through the ice faster than the speed of light. These create a shock wave (the light equmilivalent of a sonic boom), which appears as a ghostly blue glow, known as Cherenkov radiation (named after the Nobel prize-winning physicist Pavel Cherenkov).

The Cherenkov radiation is picked up by thousands of photomultipliers in digital optical modules, extremely sensitive light detectors spaced out along strings lowered through holes drilled into the ice. Because the subatomic shrapnel is emitted in roughly the same direction as the impacting neutrino, it’s possible to deduce the neutrino’s trajectory based on when the shrapnel’s Cherenkov radiation arrives at different photomultiplier.
According to IceCube scientist Mirco Hünnefeld of TU Dortmund, a university in Germany, the team faced a particularly testing problem detecting neutrinos from the Milky Way. The neutrino signal they sought is mimicked by muons, subatomic particles created when cosmic rays – high-energy atomic nuclei from space – smash into atoms at the top of the atmosphere.
“If IceCube looked through Earth, these could be screened out by the mass of the planet,” says Hünnefeld. “ But the Milky Way is in the southern sky, directly above IceCube, so no such screening was possible. It means that IceCube is hit by several thousand light-generating muons every second.”

Considerable ingenuity was required to isolate neutrinos generated in the Milky Way from this confusing background noise. Knowing that muons would predominantly interact in the surface layers of the detector, creating tracks that extend inward from the periphery, the physicists looked instead for localised cascades of particles that originated deep in the heart of IceCube.
Aided by artificial intelligence, they determined that, over a 10-year period, IceCube had picked up about 750 neutrinos from the Milky Way. “It’s quite an achievement, when you think that Milky Way neutrinos account for only about 1 in 100 million events in our detector,” says Hünnefeld.
These neutrinos were emitted from all across our Galaxy, a slender, flattened spiral of stars shaped much like a CD. “As yet, IceCube physicists can’t tell whether these neutrinos come from unknown individual sources or are instead from a diffuse background that permeates the Galactic disc,” explains Sclafani.
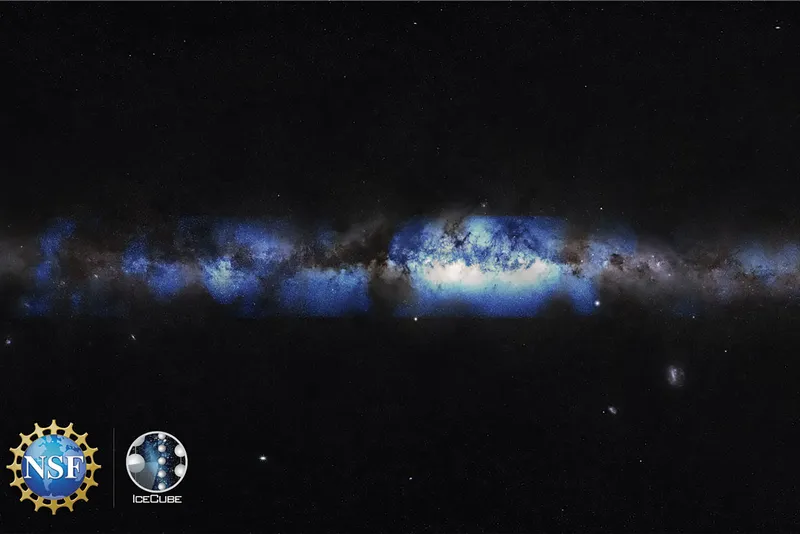
The birth of neutrino astronomy
A pedant might point out that these aren’t the first neutrinos from our Galaxy to have been detected. Since the mid-1960s, much lower-energy neutrinos from the Sun – which is, of course, in the Milky Way – have been picked up.
Higher-energy neutrinos from Supernova 1987A, which exploded in 1987 in the Large Magellanic Cloud, have also been detected – although that’s not in our Galaxy.
The crucial difference with this most recent detection is that not only is it the first time high-energy neutrinos from the Milky Way have been picked up, but it’s also the first time that a ‘map’ of their Galactic distribution has been created. This is what justifies the claim that their detection marks the birth of neutrino astronomy.
One reason why the detection of these neutrinos is important is because they could tell us about mysterious cosmic particle accelerators, which can boost cosmic rays to phenomenally high energies beyond anything achievable through human technology.
Cosmic rays can’t provide such information. Being electrically charged, their trajectories are bent so wildly by magnetic fields in space that, by the time they arrive on Earth, it’s impossible to tell where they came from.
“By contrast, neutrinos travel in straight lines,” says Sclafani. “And they can be created when cosmic rays slam into atomic nuclei close to their source. They therefore point back directly to the engine that creates cosmic rays.”
Already, IceCube scientists have traced high-energy neutrinos back to two galaxies beyond the Milky Way: NGC 1068 (aka the Squid Galaxy) and TXS 0506+056. “It’s a strange thing that we detected neutrinos from far beyond the Milky Way before neutrinos from our Galaxy,” says Hünnefeld.
“The explanation for this is that those two galaxies are prodigiously energetic objects, powered by supermassive black holes far more massive and far more active than Sagittarius A*, the one that lurks at the heart of the Milky Way.”
The details of how super-energetic cosmic rays are accelerated remain unknown. However, a general mechanism was proposed by Italian-American physicist Enrico Fermi in 1949. According to him, atomic nuclei (mostly hydrogen nuclei) bounce back and forth like ping-pong balls between shock fronts in interstellar gas, gradually gaining energy.
Such shocks are thought to exist in the expanding debris of supernovae and in pulsar-wind nebulae – basically, supernova remnants energised by a hyper-compact, spinning stellar relic of the explosion known as a pulsar.

Shock fronts are also expected to exist in the high-speed ‘jets’ shooting from the poles of the supermassive black holes that power galaxies such as NGC 1068 and TXS 0506+056. “In the case of TXS 0506+056, a violent galaxy known as a ‘blazar’, the jet is pointing directly at us,” says Sclafani. “So collisions between accelerated particles and nuclei in the local environment create neutrinos beamed in our direction.”
It’s thought that the neutrinos detected from the Milky Way come from sources such as supernova remnants and pulsar-wind nebulae, and from a diffuse background. Such a background is expected to be created by cosmic rays trapped by the Galactic magnetic field. The rays circulate in the disc of the Milky Way until they collide with an atomic nucleus in the gas and dust of the interstellar medium, creating high-energy neutrinos.
Such a neutrino background was predicted when astronomers observed a diffuse background of high-energy light coming from the Milky Way. They postulated that the light was caused by collisions between cosmic rays and atomic nuclei in the interstellar medium.
“High-energy neutrinos, such as the ones IceCube has now detected, would be an inevitable by-product of such collisions,” says Hünnefeld.
The IceCube physicists hope to pinpoint more precisely the origins of the Milky Way neutrinos when their experiment gets an upgrade, scheduled to happen in the winter of 2025/26. There are also longer-term plans to expand IceCube’s target volume to 10km3 of ice, and to incorporate new and more-sensitive photomultipliers.
In addition, the physicists hope to be able to detect not just Cherenkov radiation, but also radio waves from the cascades of particles created by neutrino collisions in the ice. The subatomic shrapnel created by the impact of a neutrino is skewed in terms of electric charge, because negatively charged electrons survive while positively charged positrons are quickly annihilated when they encounter particles of matter. The resultant fluctuating electric field creates radio waves – providing another potential avenue for detecting neutrinos.

A new way of seeing
Sclafani is excited about the future of neutrino astronomy. “We’re the new kids on the block,” he says. “It’s like the early radio astronomers or gamma-ray astronomers, who had only really blurry vision. Now they have hugely sharpened their vision and can see a multitude of discrete celestial sources. That’s what we want to achieve, too.”
According to Sclafani, neutrino astronomy has huge potential. The environments close to the supermassive black holes that are responsible for accelerating cosmic rays in galaxies such as NGC 1068 and TXS 0506+056 are clogged with dense and turbulent super-hot matter.
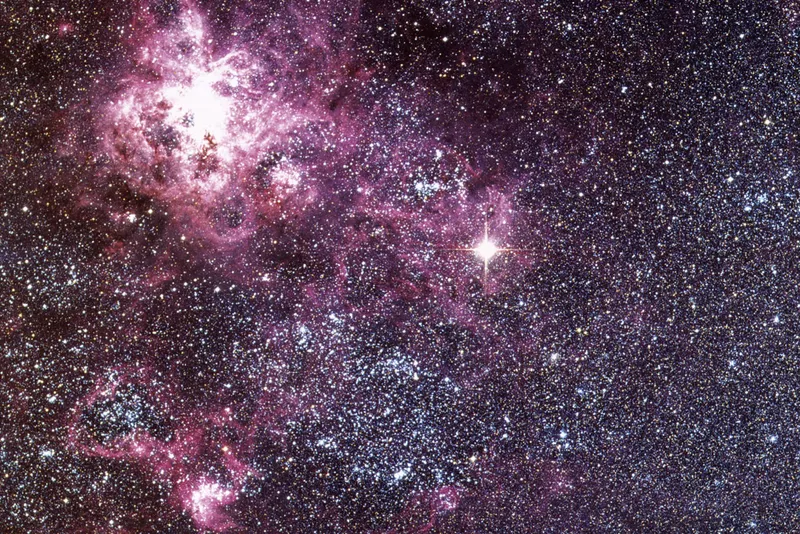
“Light is blocked and simply can’t get out, whereas neutrinos are unhindered and come straight to us,” says Sclafani.
“They represent the only way we can get information about conditions very close to supermassive black holes.”
Hünnefeld agrees that the discovery marks a major milestone in astronomy. “It opens an entirely new window on the Universe,” he says. “Now we have really big neutrino telescopes, we’re starting to make rapid progress. It’s truly an exhilarating time.”
Read more:
- Neutrinos: The vital clue to a 'theory of everything'
- What is antimatter, and why is it missing from the Universe today?
- How new antimatter science could soon explain the existence of everything
About our experts
Mirco Hünnefeld of Technische Universität Dortmund is one of the physicists involved with the IceCube Neutrino Observatory.
Steve Sclafani of the University of Maryland, is one of the scientists working on the IceCube neutrino-detection project.