Look up at the sky after sunset and the familiar quilt of night is punctured with bright stars. These blazing furnaces are so vivid that we can see their light, despite the fact that even the nearest are quadrillions of kilometres away.
It’s a sight most of us have seen on countless occasions, so you’d be forgiven for thinking that all stars must behave this way. After all, isn’t shining just what a star does?
Yet if a flurry of recent findings is to be believed, there’s an entirely different class of stars lurking out there – stellar ghosts cloaked under a veil of darkness. These transparent, invisible stars give out no light whatsoever, meaning they skulk unseen in the celestial shadows.
Astronomers already suspect that, unlike ordinary stars, most of the Universe is hidden from view. When they look at galaxies, such as our own Milky Way, they find stars on the outer edges moving far too fast. So fast, in fact, that they should fly off into space.
For them to be kept in tow, there has to be something reining them in. The most popular explanation is that there’s a lot of hidden material in the Galaxy providing a significant amount of extra gravity. Scientists call this material ‘dark matter’ and it’s thought to outnumber the ordinary matter that you and I are made of by a ratio of more than five to one.
The majority verdict over the last couple of decades has been that this celestial glue is made of Weakly Interacting Massive Particles (WIMPs). This had led physicists on an unprecedentedly intense hunt to snare them. They’ve built detectors under the ice in Antarctica, in abandoned gold mines and even aboard the International Space Station.
So far all their searches have come up empty. It’s somewhat ironic, then, that one of our WIMP detectors may have just found evidence in favour of a rival theory of dark matter – one that opens the door to the possibility of invisible stars.
Read more about dark matter:
- What is dark matter?
- Desperately seeking dark matter: the search for 95 per cent of the Universe
- Could ‘dark matter’ just be dead stars and planets floating in the depths of space?
Dark bosons
The XENON1T experiment is tucked away 3,600 metres beneath the Gran Sasso mountain in Italy and is the largest underground research facility in the world. A huge tank containing over three tonnes of liquid xenon was designed to act as a WIMP trap – if a WIMP hits an atom in the tank, then the atom will recoil and spit out electrons and photons (particles of light).
Yet in the summer of 2020, the XENON1T researchers announced that they’d seen something unexpected: an excess of electrons that didn’t fit with an influx of WIMPs. According to Dr Tongyan Lin, from the University of California, San Diego, there are three possible explanations.
The first two explanations are particles from the Sun, or radioactive contaminants in the experiment. The third, and by far the most interesting, is the arrival of another proposed form of dark matter: dark bosons.
A boson is a subatomic particle that carries a force. The photon, for example, is a boson that carries the electromagnetic force. A dark boson, so the theory goes, could either be dark matter itself or at the very least be responsible for the way dark matter interacts with ordinary matter. If the XENON1T signal stands up to further scrutiny – and the other more mundane explanations can be excluded – it could be the first sign that dark bosons are indeed out there.
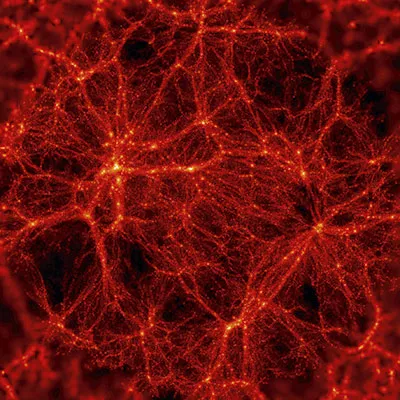
A further tantalising hint followed in September 2020, a few months after the XENON1T announcement. Two teams of physicists – one in Europe and the other in the USA – used lasers to confine atoms in a table-top trap. Like all atoms, they contained electrons whizzing around a central nucleus in orbits known as energy levels.
Dr Michael Drewsen, from Aarhus University in Denmark, is part of the European team. He says that the presence of a dark boson would create a force that disturbs the atom. “We’d see a small shift in the electron’s energy level,” he says.
While his team didn’t find such a shift, his colleagues in the USA did. As always, scientists are a cautious bunch and aren’t able to immediately leap to the conclusion that a dark boson really is to blame.
“It could be because they were using a heavier atom,” Drewsen says. The European team trapped calcium, whereas the American team used ytterbium. Still, their findings, coupled with those from XENON1T, are a shot in the arm for those arguing dark bosons are real. The circumstantial evidence is certainly mounting.
Astronomers are bolstering the case yet further. If dark bosons are affected by gravity, then they should also clump together in the same way that ordinary matter does. “They would self-gravitate into boson stars,” says Hector Olivares, from Radboud University in the Netherlands.

These stars would be very different from those strung out in constellations across the night sky. For starters, with no nuclear fusion taking place in their cores, they wouldn’t produce any light. They would also be transparent. “Anything that approached them would pass straight through,” says Olivares.
The lack of any non-gravitational interaction between ordinary matter and dark matter means it would be like a ghost drifting through a wall. After all, the only reason you don’t fall through a chair is the repulsive electromagnetic force between the electrons in your bottom and those in the seat.
According to Olivares, a boson star could potentially grow as big as the supermassive black holes (SMBHs) thought to reside at the heart of every major galaxy. In fact, he suspects it may be possible for a giant boson star to initially fool us into thinking it’s a SMBH. “Both of them lack a solid surface,” he says, referring to the fact that a black hole is a cosmic trapdoor with a point of no return known as the event horizon.
Black holes and dark boson stars
Olivares recently conducted the first simulations of material falling towards a black-hole-like boson star. “We discovered that they are distinguishable from black holes,” he says. That’s because they lack a shadow.
In 2019 astronomers released the first-ever image of a black hole, including a dark region – a shadow – rendered by the missing light that the black hole swallowed. While a boson star doesn’t have a shadow – material passes straight through instead of being swallowed – it does sometimes have a feature that does a good job of impersonating one. Olivares calls it a pseudo-shadow.
“In most cases we don’t see a pseudo-shadow and when we do it’s smaller than a black hole’s shadow,” he says. We could soon use this as a test to see if the SMBH at the centre of the Milky Way is actually a giant boson star. “It’s something that can be distinguished using the Event Horizon Telescope [which was the same instrument used to capture the first black hole photograph],” Olivares says. That work is currently ongoing.
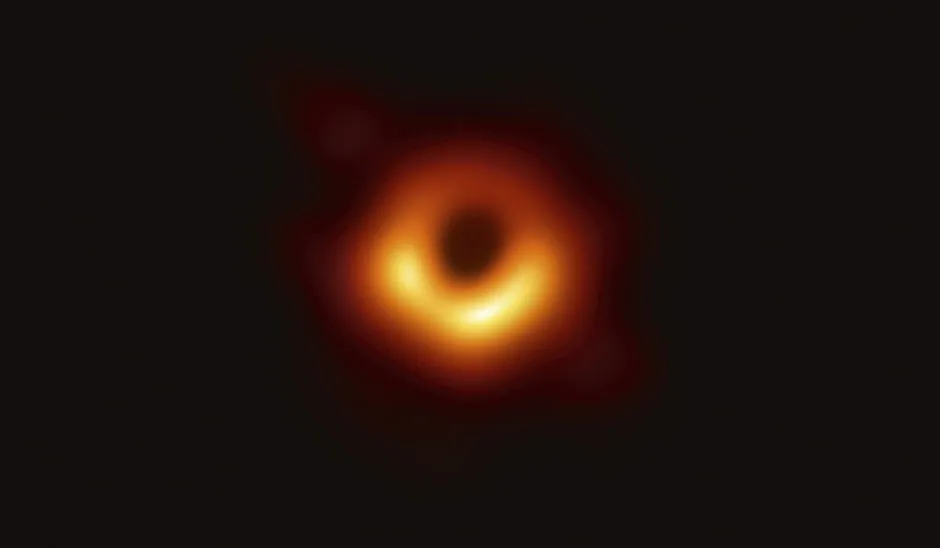
While we patiently wait for that result, Dr Juan Calderón Bustillo from the University of Santiago de Compostela in Spain may have already found two boson stars masquerading as black holes. Calamitous celestial collisions create ripples – gravitational waves – which trundle out through the Universe and reach Earth.
They were picked up for the first time back in 2015 using the Laser Interferometer Gravitational-Wave Observatory (LIGO) in the USA. The majority of the events we’ve seen so far have been binary black holes – two gravitational monsters orbiting each other before spiralling into oblivion.
Usually, there are three distinct stages to such a collision – the in-spiral, the merger and then the new mega black hole it creates. But, according to Bustillo, one particular event sticks out as odd: GW190521. “We don’t see that first in-spiral stage,” he says. “It could be a head-on collision instead.”
The rest of the black hole mergers we’ve seen so far come from two black holes already orbiting each other. However, if two previously unconnected black holes smashed together, that could explain the lack of an in-spiral stage before collision. So Bustillo did the maths, but that explanation didn’t fly.
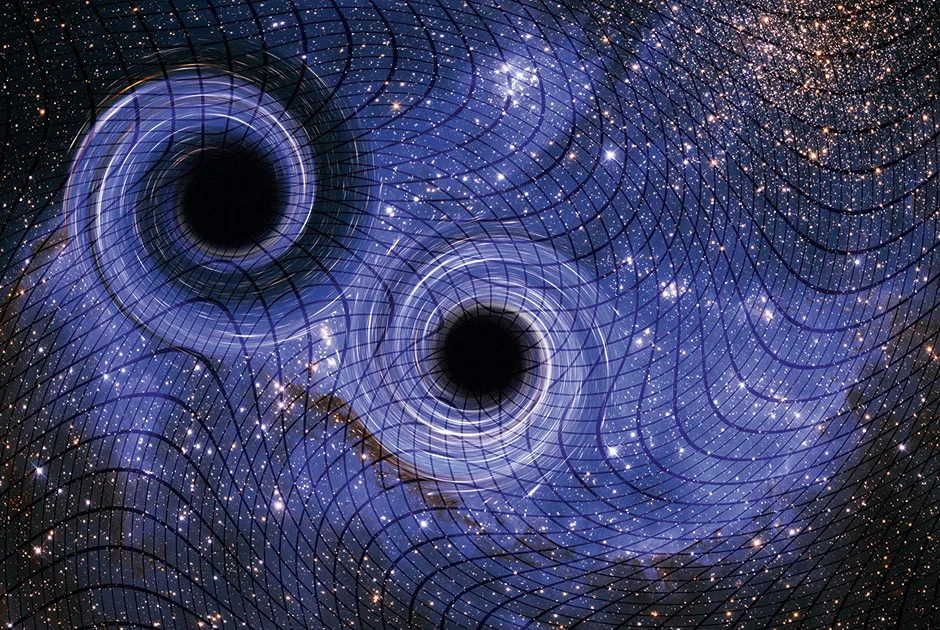
“The gravitational wave signal lasts longer than you would expect,” he says. The resulting black hole also spins faster than it should – a head-on collision wouldn’t provide the same rotational boost as a pair of black holes already pirouetting around one another. “So the gate is open for other explanations,” he adds.
Bustillo wondered if a head-on collision between two boson stars could fit the bill instead. It turns out they can. According to his research, there’s an extra stage in the process for colliding boson stars, compared to colliding black holes. The big boson star created from the two colliding ones oscillates for a bit before becoming a black hole.
This extra oscillation stage could explain why the signal lasted longer than you’d expect for two colliding black holes. Bustillo was also able to use the collision data to calculate the mass of the bosons making up the stars. “The value is around the current constraints from other measurements,” he says. In other words, it fits with our existing ideas about dark matter.
The real clincher will come as we see more gravitational waves from collisions without an initial in-spiral stage. “I do expect the detectors to see more signals like this,” Bustillo says. If they can also be explained by colliding boson stars, and each independent event consistently gives the same mass for the dark bosons, then it’ll get harder to ignore the possibility that see-through stars are out there.

Two upcoming experiments could soon join the fray and help us to shore up the case further, according to Dr Costantino Pacilio from Sapienza University of Rome. The first is the Einstein Telescope, a proposed European ground-based gravitational wave detector. The second is the Laser Interferometer Space Antenna (LISA), a trio of spacecraft that will fly in formation separated from each other by 2.5 million kilometres.
“They will both have a higher sensitivity than LIGO, meaning we will get a more accurate and detailed look at the shape of the gravitational waves,” says Pacilio. That’s crucial, because every colliding object imprints its features into the shape of the waves.
In particular, the way the two colliding objects deform each other with their gravity provides a unique signature. “Boson stars are exotic objects,” Pacilio says. “They only interact gravitationally with the Universe, so this is the only way they can show themselves.”
When we invented the telescope, it was to get a better view of the things we could already see. But now, centuries later, it’s becoming increasingly apparent that there’s a lot more to the Universe than meets the eye. Perhaps it’s time to turn our ideas about stars upside-down and accept the fact that there could be just as many invisible stars creeping through the Universe largely unseen.
- This article first appeared inissue 360ofBBC Science Focus Magazine–find out how to subscribe here