Physicists are homing in on the mass of the neutrino, nature’s most elusive subatomic particle. The latest super-accurate measurement, made by an experiment in Germany, shows that the neutrino is around half a million times less massive than the electron, the lightest particle of normal atomic matter.
According to the Standard Model, the high point of 300 years of physics which describes the fundamental building blocks of matter and three non-gravitational forces that glue them together, the neutrino should be massless.
So why should we care about a mass measurement (no matter how tiny) of a neutrino? Well, it may provide vital clues to the fabled ‘theory of everything’ – a deeper, more fundamental theory of physics of which the Standard Model is believed to be but an approximation.
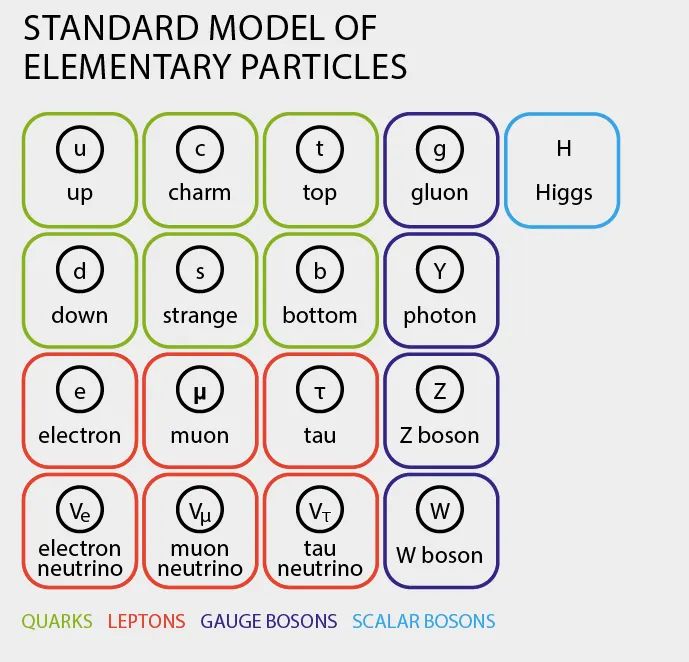
Hunting the elusive ghost particle
The latest neutrino measurement was made in Karlsruhe, Germany, where physicists exploited the ‘beta decay’ of tritium. Tritium is a heavy type – or ‘isotope’ – of hydrogen. In beta decay, the unstable core – the ‘nucleus’ – of an atom sheds surplus energy by spitting out an electron and an antineutrino (the neutrino and its ‘antimatter’ twin have the same mass).
Neutrinos are fantastically antisocial, interacting so rarely with normal matter that they could pass unhindered through several light-years of lead. Consequently, the physicists at the Karlsruhe Tritium Experiment, or KATRIN, must infer the neutrino mass from measurements made on their electrons.
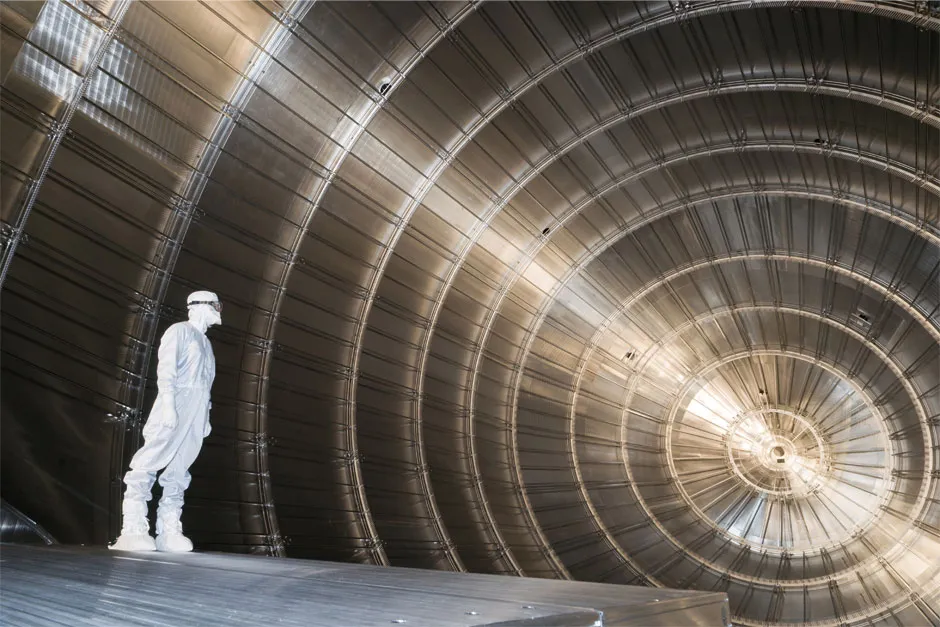
They can do this because the amount of energy emitted by the tritium nuclei is always the same. The energy is divided between the electron and the neutrino – if an electron has lots of energy, then it must mean that its associated neutrino only has a little bit. So if the physicists only allow the most energetic electrons to reach their detector, it ensures that their associated neutrinos will have very little energy – this allows them to make a more accurate reading of the neutrinos’ mass.
Read more about the Standard Model:
- The weak nuclear interaction: the enigmatic fundamental force that makes life possible
- Who really discovered the Higgs particle?
- Wolfgang Pauli and the discovery of the Universe's most elusive particle
KATRIN is an extraordinary piece of engineering. After 18 years of planning and building, it weighs 200 tonnes and cost about €50m (£42m). It is operated by a team of 150 people from six international institutions, and yielded its first result after only one month of operation after observing two million electrons.
The experiment found that the neutrino cannot weigh more than 1.1eV (because Einstein showed that mass is a form of energy, physicists measure the masses of subatomic particles in energy terms – an eV is an electron volt).

By comparison, an electron has a mass of 500,000eV. “The result is an incredible achievement,” says Dr Melissa Uchida, a neutrino physicist at the University of Cambridge. “The uncertainty in the mass limit is 100 times better than the previous best estimate.”
There is a twist to this story – a major one. The electron-neutrino is merely one of three types, or ‘flavours’, of neutrino. The electron-neutrino is associated with the electron, but there is also the muon-neutrino associated with the heavier ‘muon’ particle, and the tau-neutrino with the even heavier ‘tau’ particle.
There are three distinct mass states of the neutrino. But, crucially, each does not correspond to a flavour – in fact, each neutrino is a different mix of all three masses. Imagine an animal that is 25 per cent cat, 25 per cent dog and 50 per cent giraffe.
This conveys some idea of the weirdness of neutrinos. As each type flies through space, its individual mass components travel at different speeds, and consequentially the relative proportions of each mass state changes. This results in a neutrino ‘oscillating’ between an electron-, muon- and tau-neutrino.

Measurements of neutrino oscillations will provide estimates of the differences between masses of the three neutrinos. Importantly, KATRIN pins down an upper limit on one mass. Crucially, however, we still don’t know the neutrino-mass hierarchy – whether electron-, muon- and tau-neutrinos get progressively more massive as do electrons, muons and taus.
Understanding neutrino oscillations and neutrino masses is vitally important. If the ‘mixing’ between neutrino mass states is big enough, it could indicate that nature permits a process that, in the jargon ‘violates charge-parity symmetry’. This would make antineutrinos behave differently from neutrinos.
By favouring the production of matter over antimatter, this could solve one of the outstanding puzzles of cosmology: why we live in a Universe of matter. “According to the Standard Model, all fundamental particle processes create equal quantities of matter and antimatter,” says Uchida. “We therefore should not exist [because when matter and antimatter particles meet, they annihilate]!”.
Rethinking the early Universe
Neutrino oscillations may reveal the existence of a fourth, ‘sterile’ neutrino, interacting with matter so rarely it makes the other three flavours appear positively sociable. The total mass of all three (or more) types of neutrino has consequences for the Universe because neutrinos are the second most common subatomic particle, after photons. In the early Universe, their considerable gravity would have helped matter clump together to make the first galaxies.
The more massive neutrinos are, the earlier they would have slowed down after the Big Bang and the clumpier our Universe should be. Consequently, knowing the masses of the neutrinos helps pin down the cosmological model that best describes our Universe. If astronomers’ observations of clumpiness contradict that model, then it will be strong evidence of physics beyond the Standard Model.
- This article first appeared in the March 2020issue ofBBC Science Focus Magazine