A new 3D reference atlas of the mouse brain will enable researchers to zoom down to the level of a single cell. By studying the mouse brain’s structure in such detail, neuroscientists will be able to advance their understanding of how the mouse brain functions, and how this functioning can go wrong.
The atlas – called the ‘Allen Mouse Brain Common Coordinate Framework’, or ‘CCFv3’ – was created by researchers at the Allen Institute for Brain Science in Seattle, USA.
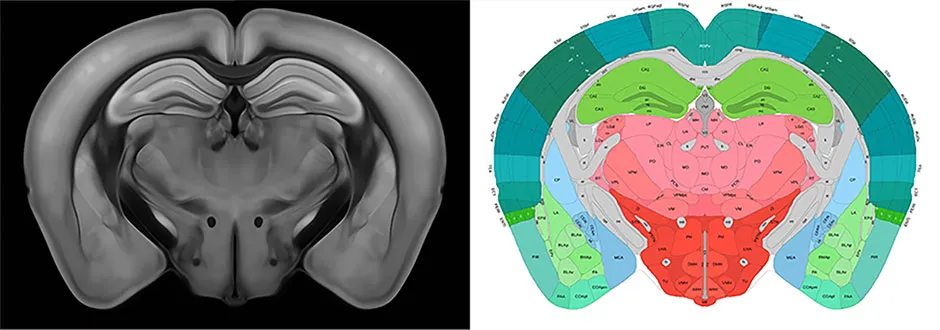
They averaged the brain scans of 1,675 mice, made using a technique called ‘serial two-photon tomography’. This works by shining a laser on the brain tissue and measuring how the tissue emits light, or ‘fluoresces’, in response.
Each point on the atlas’s 3D grid was labelled according to the brain structure it belongs to. In total, the researchers labelled more than 800 structures, including several that hadn’t featured in previous mouse brain atlases.
Read more about brains:
- Brain cells’ ability to work as a team strengthens memories
- Exercise and the brain: why moving your body matters
Brain atlases are useful for neuroscientists because they provide a reference when studying brain disease. For instance, this atlas will allow researchers to compare the 3D volumes of different brain structures in healthy and diseased mouse brains.
This could in turn lead to insights into the human brain, as mice are often used as a model for understanding human neurological disease – partly because our genomes are so similar (around 90 per cent of genes associated with disease in mice have counterparts in humans).
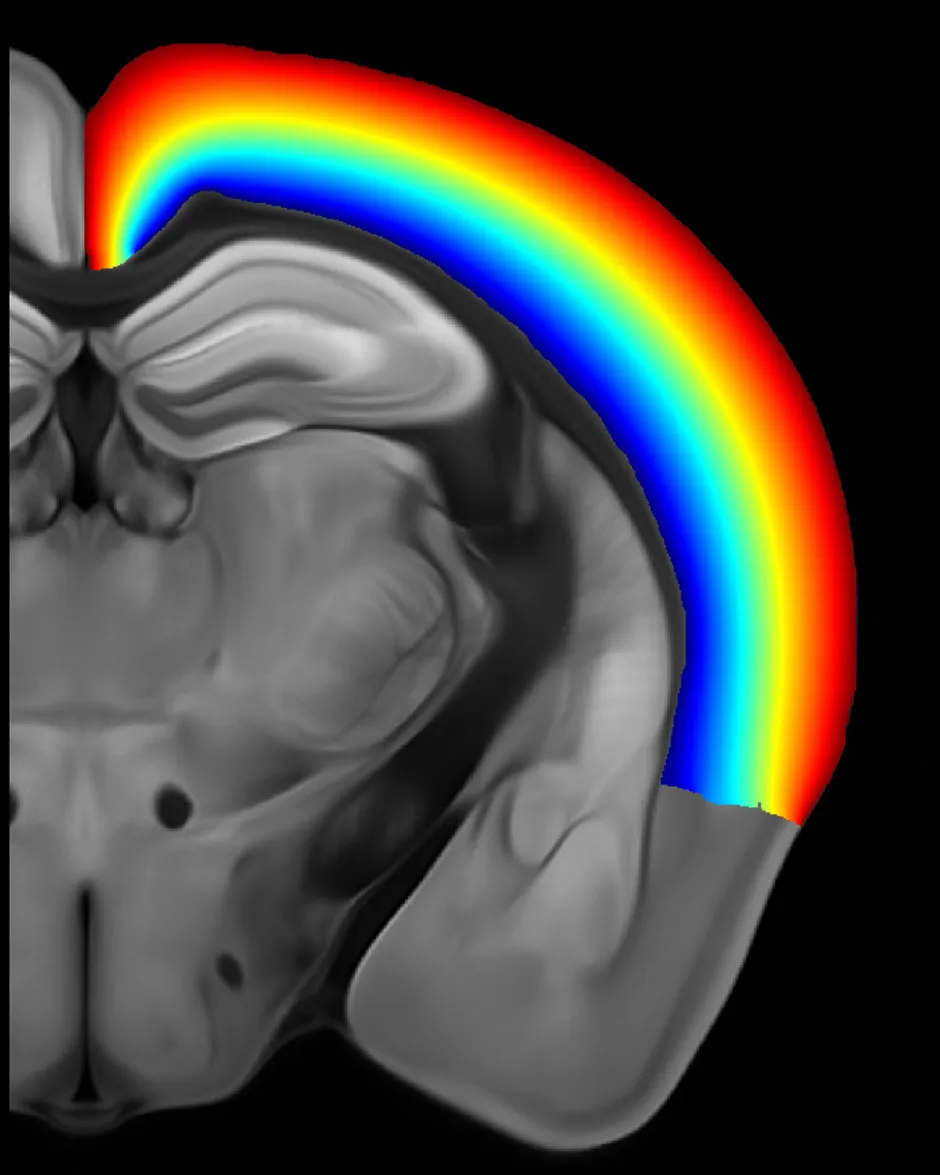
“We hope the wider neuroscience community will use it as a new standard reference atlas,” said co-senior author of the study Dr Lydia Ng.
“By making our atlas and related tools open access, new data and data types generated across our community can be more easily integrated and compared in the same spatial context, and the atlas in turn can be modified as our knowledge about brain structure evolves.”
How does an MRI scanner work?
Magnetic Resonance Imaging (MRI) takes advantage of the fact that the nucleus of a hydrogen atom (a single proton) behaves like a weak compass needle. In the presence of a strong magnetic field, the hydrogen atoms will align themselves, but a radio signal of the correct resonant frequency will cause them to deflect slightly.
When the signal is removed, the atoms return to their equilibrium state and emit a radio signal of their own. An MRI scanner can detect these signals and use them to map the distribution of molecules with lots of hydrogen atoms – ie, water and fat. In this way, it can create detailed images of the inside of the body.
Scanning table: The patient can only be scanned from inside the magnetic coil, so a motorised table slides them in and out.
RF system: An antenna produces a radio signal to ‘nudge’ the hydrogen nuclei and listen to the answering radio wave they emit.
Liquid helium: Liquid helium is pumped through an enclosing jacket to cool the superconducting magnets almost to absolute zero.
Main magnet: Superconducting magnetic coils produce a magnetic field of 1.5 teslas – that’s about 300 times stronger than a fridge magnet.
Patient: The high magnetic fields mean that patients with cochlear implants, pacemakers or embedded shrapnel usually can’t be scanned.
Gradient system: A second coil distorts the main magnetic field so that the resonant frequency of the protons varies according to position.
Read more: