Whenever there's big news in the world of physics, most of us react with a curious mix of nerdy excitement and utter bewilderment at the weird, head-spinning details. That's exactly what happened yesterday. Researchers at Fermilab near Chicago announced the results of an experiment suggesting a brand new force of nature could be at work in their findings.
An international team of physicists were studying the behaviour of short-lived subatomic particles called muons. They sent them hurtling around a particle accelerator and watched closely what happened when a magnetic field was applied. The short explanation is that they didn't do what they were supposed to do.
The Standard Model of particle physics, the best blueprint we have for understanding the building blocks of reality, predicts the muons should have 'wobbled' in a certain way when in fact they wobbled in a different way. This confirmed another decade-old finding and got physicists everywhere – but particularly on Twitter – extremely excited.
Why? Because if it's not in the Standard Model, then it implies something brand new in our understanding of the Universe. It could be a new sub-atomic particle, like the Higgs Boson, discovered to much fanfare in 2013. Or it could be a new fundamental force of nature (brilliantly called the flavour force), which would sit alongside gravity, magnetism and the strong and weak nuclear forces that affect sub-atomic particles.
Either way, it's new, it's freaky and trying to wrap your head around it is potentially migraine-inducing. Which is why we called up Professor Jon Butterworth, particle physicist at UCL who conducts experiments with the Large Hadron Collider in Switzerland. Here's his bluffer's guide to muons and how they could expand our understanding of, well, everything.
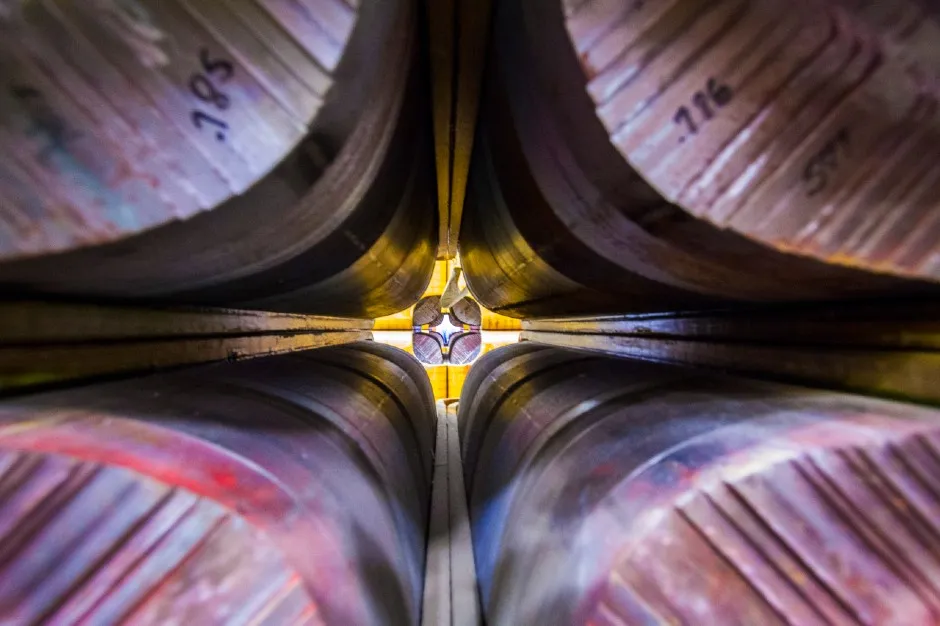
What are muons?
The muon is essentially the same as a regular electron apart from the fact that it's heavier. It's got more mass. They're not new in and of themselves. They were first seen in cosmic rays – high energy particles in the upper atmosphere – for the first time a long time ago – early to mid-20th Century. So we've known about them for a while.
This experiment particularly looked at them from a magnetic perspective, right?
Yes. All particles, including muons and electrons, carry angular momentum. They're spinning. That gives them what we call a magnetic dipole – a north and south pole, basically. And it's the interactions of that dipole that they've been studying. The way you characterise dipoles is called a magnetic moment: it's just, how big is the magnetic field a thing has because it's spinning? The muon's is the wrong size. That's the long and short of it.
You can calculate that dipole really precisely in our Standard Model of physics. In fact, doing that for the electron was one of the benchmarks, a kind of poster child for how amazing the precision of this theory really is. They're basically trying to reproduce that for the muon. Both the measurement and the calculation are super precise but they don't quite agree and this experiment confirmed that discrepancy, basically.
And that implies there's a new force of nature?
Yeah... Well, we don't really know what it implies, but taken at face value it means that something's going on that's not in the Standard Model, which means a new force or a new particle.
Is this new force – if it is a new force – likely to have any tangible effect on the way we experience the world?
It's unlikely to show up in everyday life. It will have an effect on things we observe with our science. Our understanding of the fundamental constituents of nature leaves a few things unanswered. One of the things it leaves unanswered is why there isn't an equal amount of matter and anti-matter in the Universe. It seems to be mostly matter, but the forces are completely symmetric.
What we do when we try to pick holes in the Standard Model and look for anomalies like this is we're trying to look for clues to these things that the Standard Model doesn't answer. So this may help to answer that. And so, from one point of view, that's quite a big impact on everyday life because it might explain why we have everyday life! On the other hand, it's not going to be something that makes a new car or something tomorrow.
Read more about particle physics:
- Do subatomic particles have a colour?
- What's the most abundant particle in the Universe?
- The particle detectors probing the Universe
Why is it called the flavour force?
Flavour is the name we give to the difference between electrons and muons. The Standard Model comes in three 'generations' – there's an electron, a muon and a tau, which is even heavier than a muon. The flavour is the difference between them, so the reason it's called the flavour force is that this effect is clearly affecting the electron differently to the muon. It depends on the flavour.
There was a fuss just a couple of weeks ago from CERN and the LHC, which was also hinting that there was some difference between electrons and muons. That was a completely different measurement, nothing to do with magnetic moment, but the decay of a rare particle containing a bottom quark. What was interesting was again, the Standard Model said that electrons and muons should behave the same, and in this case they didn't.
People are getting excited that these straws in the wind are all blowing in the same direction, in the same wind. And that wind is some kind of flavour force. A force that depends on flavour.
Was this something that physicists were actively hunting, like the Higgs Boson?
Not really. In one sense it's the same in that there's a prediction in the Standard Model are you go and see if it's right. That's basic science. The Standard Model predicted that Higgs would be there so we went and looked for it. The Standard Model also predicts what the magnetic moment of the muon should be, very precisely, so we go and check that prediction. That's what's happened here.
The difference with the Higgs is, the Standard Model was right and we found it. In this case, it's not a completely new thing but it's not agreeing with what the Standard Model predicts. So in that sense, it's more exciting.
Read more about physics:
- Six mind-blowing physics lessons with Jim Al-Khalili
- The seven biggest mysteries in the Universe
- The six numbers that define the entire Universe
Is the Standard Model in trouble then? What are the consequences of that?
Well, we all hope it is in a sense. We know the Standard Model is an effective theory but we also know that it's not the ultimate theory because of these things it doesn't explain, like the matter/anti-matter thing. Whenever we see something that doesn't quite fit with the Standard Model, we're hoping it will be a clue to a better theory – one that will still include the Standard Model.
The analogy that people often use is Einstein and Newton. Newton's laws of force and motion weren't completely wrong. They're contained within Einstein's relativity. We think the same is true of the Standard Model. It's an effective theory that obviously works really well for everything we've measured so far. What we hope is that it will lead to another model that explains some of the things that the Standard Model doesn't.
So it's good news.
Definitely good news. We like this kind of thing because it's when science progresses. Isaac Asimov said, 'The best phrase in science is not Eureka, it's, Hm, that's funny.' This is definitely a that's-a-bit-funny moment. We've had this anomaly for 10 years and it's just been confirmed by another experiment. It's growing in its funniness, shall we say.
It's not brand new then?
This measurement is brand new but another measurement was made about a decade ago which came up with the same kind of discrepancy. One measurement is never enough. They had to move the equipment from Long Island to Chicago, where they could do a better measurement, basically because they've got more muons. This is an independent measurement and gives the same result, and that's why people are getting more excited. And over the next few years, it will get repeated and the measurement should get more precise. More muons basically, because muons decay it's quite hard to catch them and store them.
Is anyone jumping to conclusions yet about what this might be?
Oh yeah. Theorists always jump to conclusions! The trouble is, everyone jumps to different conclusions so there's no consensus yet. It's not like when we saw a bump and everyone thought, 'That's the Higgs Boson.' There are loads of pet theories flying around this morning.