ProtoDUNE, French-Swiss border

Detecting: Neutrinos
Bathed in yellow light to avoid overstimulating its sensors, this neutrino detector is the size of a three-storey house. Neutrinos are abundant – trillions pass through you every second – but they hardly interact with matter, making them tough to detect. When in operation, ProtoDUNE is filled with 800 tonnes of liquid argon. Sometimes, a neutrino makes a direct hit on an argon nucleus, producing a trail of charged particles detected by grids of wires around the detector.
This prototype is being tested at CERN’s headquarters, but DUNE (Deep Underground Neutrino Experiment) will be sited 1.5km underground in the disused Homestake gold mine in Lead, South Dakota. With four detectors, DUNE will pick up neutrinos generated by a particle accelerator 1,300km away at Fermilab, near Chicago. Expected to go live in 2026, DUNE will detect differences in behaviour between neutrinos and their antimatter counterpart, antineutrinos, which could help explain why the Universe has more matter than antimatter.
Read more:
- The masters of natural disasters
- CERN’s trigger system: how the LHC copes with a constant flood of data
HAWC(High-Altitude Water Cherenkov) Observatory - Mexico
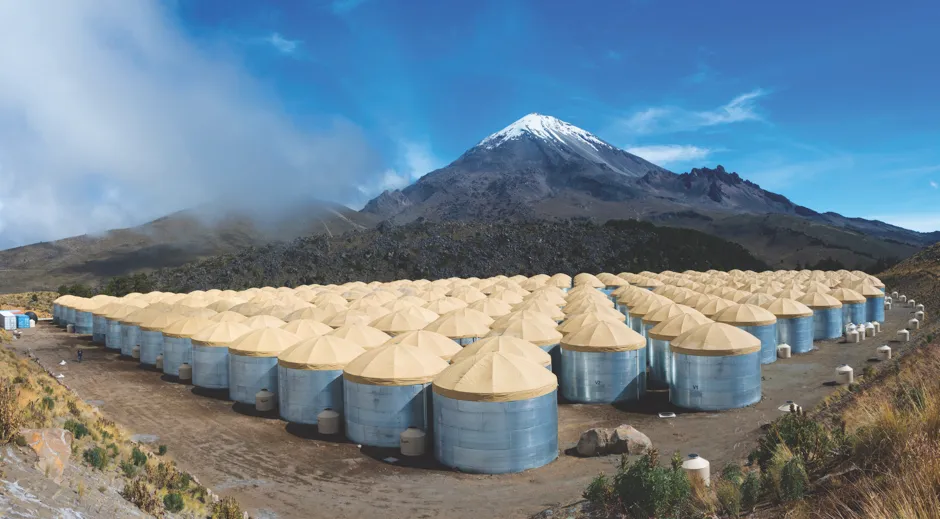
Detecting: Gamma rays
In the shadow of Mexico’s Pico de Orizaba volcano, these 300 steel tanks make up the HAWC, which is on the lookout for gamma rays – high-energy radiation that’s created by cataclysmic events in space. When gamma rays hit our atmosphere, they produce a shower of fast-moving particles, which can interact with water molecules to create ‘Cherenkov radiation’, visible as an eerie glow.
Each of the seven-metre-wide tanks is filled with water, plus detectors for picking up the radiation. By comparing the times at which particles arrive in the tanks, it’s possible to work out the direction of the gamma rays. HAWC has recently been used to study the SS 433 microquasar, around 15,000 light-years away. SS 433 consists of a black hole consuming a star, pushing out jets of matter that generate gamma rays.
LIGO (Laser Interferometer Gravitational-Wave Observatory) - USA

Detecting: Gravitational waves
This 40kg, red-tinted mirror is suspended by an elaborate system designed to protect it from vibrations. This means that it’s capable of detecting movements less than the size of an atomic nucleus, indicating the arrival of gravitational waves. Comprising two sites over 3,000km apart, LIGO is the first successful gravitational wave detector. Each LIGO site has a pair of four-kilometre-long tubes, which lasers repeatedly travel through, revealing movements in these mirrors.
On 14 September 2015, astronomy was changed forever by LIGO’s first detection of gravitational waves. Predicted by Einstein in 1916, these vibrations in the fabric of space-time are caused by cosmic collisions like merging black holes. So far, LIGO has detected 11 black hole and neutron star mergers, and is just starting a third run.
Read more:
Super-Kamiokande - Japan

Detecting: Neutrinos
Over 13,000 light-detecting tubes line the Super-Kamiokande neutrino detector, one kilometre beneath Mount Ikeno in Japan. This is the granddaddy of such detectors, beginning operations in 1996. The 40m-wide tank holds 50,000 tonnes of pure water. When neutrinos collide with the water molecules, they produce fast-moving electrons, which generate Cherenkov radiation, detected by the tubes in this photo.
Super-Kamiokande has been central to our understanding of neutrinos’ weird behaviour. We know that the Sun produces vast quantities of neutrinos, but only about a third of them were being detected. Super-Kamiokande, along with Canada’s Sudbury Neutrino Observatory, was used to show that neutrinos go through a process called oscillation, shifting between three different types in flight – which explained why so many were going undetected. This showed that – contrary to expectations at the time – neutrinos have mass, flagging up a gap in our understanding of how the Universe works.
Deap-3600 (Dark Matter Experiment using Argon Pulse-shape) - Canada

Detecting: Dark matter
This flower-like array of photomultiplier tubes in the DEAP-3600 detector surrounds a chamber of liquid argon. The tubes are pointed inwards, looking for tiny flashes of light as incoming dark matter particles interact with the argon nuclei. If neutrino detectors have a tough job, at least we know what we’re looking for. Dark matter, on the other hand, has proved trickier to pin down.
This exotic matter – predicted by measurements that galaxies seem to have much more matter in them than is observed – should outnumber ordinary matter particles by around five to one. One theory is that dark matter is made of WIMPs (‘weakly interacting massive particles’), and these are what DEAP-3600 is designed to look for. To reduce interference from other particles, the detector is located two kilometres underground in an old nickel mine at Sudbury, Ontario.
DEAP-3600 began operations in 2016, and the first data is just starting to be analysed. So far, nothing has been found.
- This article was originally published inBBC Science Focus in May 2019 –subscribe here
Follow Science Focus onTwitter,Facebook, Instagramand Flipboard