Take even a quick peak beneath Earth’s surface and you soon discover just how much we don’t know about what’s happening right under our feet.
We spend so much of our time focused on the world around us that we rarely give much thought to what’s going on at the core of our planet. If Earth were an apple, the crust that we live on would only be as thick as the apple’s skin.
The structure of the Earth
How hot is the Earth's core?
Like an apple, Earth also has a core tucked away within, buried beneath a layer called the mantle. The core formed early, just 200 million years after Earth itself coalesced, some 4.5 billion years ago. Earth’s core is large – almost equivalent to half the size of Mars – and there’s such extreme pressure crushing down on it that its temperature is as hot as the surface of the Sun.
To put that into numbers, that's around 6,000°C! Keep in mind, too, that Earth’s core is only around 3,000km from the surface – if the Sun were as close as that, it would melt us entirely.
What is the Earth's core made of?
There are two main parts to the Earth's core; an inner core and an outer core, followed by the mantle and the crust:
1. Inner Core
A solid, crystallised iron structure that’s under immense heat and pressure. Each layer of the crystal structure is thought to be hexagonal in shape, although there may actually be two separate crystalline structures present. The crystals are believed to align roughly north-south to match the orientation of the Earth’s rotation axis and its magnetic field.
2. Outer Core
This is the only truly liquid layer of Earth’s internal structure. Around 2,000km thick, the outer core is mostly iron and nickel, with between five and ten per cent made up of lighter elements. The transition between the inner core and outer core is located approximately 5,150km beneath Earth’s surface.
3. Mantle
Together, the crust and the top half of the mantle make up the lithosphere, which is broken into tectonic plates that shift. These shifts cause earthquakes and the continents to drift. The mantle is by far the largest part of the Earth, making up 84 per cent of its total volume.
4. Crust
The crust is split into the oceanic crust, which is a maximum of 10km (6.2 miles) thick, and the continental crust, which can be as much as 80km (49.7 miles) thick in places. The crust rises and falls by up to 25cm each day as the Moon pulls on it.
How do we know the composition of the core?
Earthquakes have played an indispensable role in our understanding of this internal structure. The modern seismometer, invented in 1880, measures the vibrations from earthquakes as they ripple through the planet. In the early 20th century, scientists assumed that Earth’s core was completely molten and the material’s movement was responsible for generating the planet’s magnetic field.
Then, in 1936, the Danish seismologist Inge Lehmann was able to determine, through the use of seismometers, that seismic waves were bouncing off something deep inside Earth. She correctly concluded that the planet’s core was composed of two parts: a solid inner core, nested Russian-doll-style, inside a molten outer core.
But more recent work is revealing that the reality could be a touch more complicated. Dr Thanh-Son Phạm and Prof Hrvoje Tkalčić from The Australian National University tried something different. “We claim the detection for the first time of ricocheting seismic waves, which propagate from the earthquake source to the other side of Earth, and back, up to five times,” Phạm says.
“The detection is significant because it allows a new way to probe the very centre of Earth, which was very unlikely in the past.” It’s a technique that’s often been used in the search for new minerals but not for probing Earth’s inner structure.
Publishing their findings in February 2023, Phạm and Tkalčić analysed data from the growing network of seismometers set up across the planet. The important part was getting data from close to the epicentre of the earthquakes and then from the exact opposite spots on the other side of the planet, known as the antipode.
The reason this has been tricky in the past is because earthquakes tend to cluster around an equatorial belt dominated by oceans and other remote areas.
When an earthquake strikes, the ensuing vibrations reverberate inside the planet for days. They take about 20 minutes to cross from one side of the Earth to the antipode. Phạm and Tkalčić saw up to five back-and-forth bounces from several magnitude-six earthquakes.
The waves got weaker with each bounce, so they used a technique called stacking to combine them. This helped the two scientists to draw out more information from the weaker signals. Only two bounces had been analysed prior to their work.
Seismic waves travel at different speeds
The scientists found that the seismic waves travelled differently through the innermost inner core than the outermost. The waves slowed down when they hit the solid core but they slowed down in different directions.
Phạm says that this suggests the crystals of iron that make up the core are arranged differently in the inner core. They estimate that the innermost inner core is 650km thick and takes up slightly more than half of the inner core.
There’s more work to be done, however. “The [question of the] nature of the transitional layer between the innermost region and the upper layer of the inner core remains to be answered,” Phạm says. “Hopefully, this question can be addressed in the near future.”
Understanding Earth's core could help us with Mars
Understanding its exact structure is important because Earth hasn’t always had a solid core – it’s believed to have formed between 600 million and 1.5 billion years ago. Insights into its structure could also help astronomers and planetary scientists to understand more about what happened to Mars.
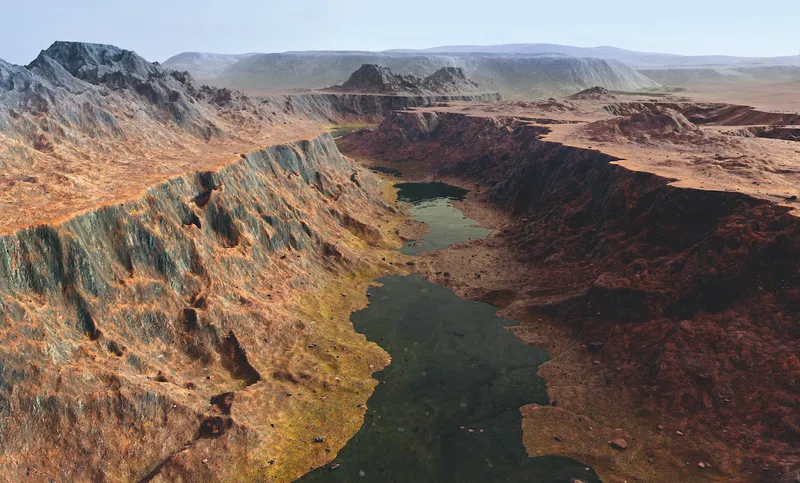
Data from Mars rovers hint at a warmer, wetter past for the Red Planet that would have made it a lot more like Earth. If Mars’s core solidified completely, its magnetic field would have switched off, leaving it unprotected from the ravages of the solar wind that gradually pecked away the majority of the Martian atmosphere.
What’s happening to its spin?
Our planet’s spin is slowing down and our days are getting longer as a result.
How long is a day? 24 hours? 86,400 seconds? The answer is no two days are ever the same. A day is defined by how long it takes Earth to complete one rotation on its axis and many factors affect the speed of our spin. The gravitational influence of the Moon has dragged on the day, lengthening it from just under 19 hours 1.4 billion years ago to the more familiar 24 hours today.
This is not only predicted by the physics of tidal forces but also backed up by studies of 430-million-year-old fossilised coral. As the coral grew, it laid down a new line of calcium each day. These lines are arranged in patterns that represent the seasons. There are 420 lines within those patterns, meaning 420 days annually. As the year is the fixed amount of time it takes Earth to orbit the Sun, more days mean fewer hours in each one and just under 21 hours at the point the coral stopped growing.

The melting of the polar caps at the end of Earth’s regular ice ages has also played a halting role. These are long-term trends. More short-term effects include an earthquake in Chile in 2010 that sped up the planet and shortened the day by 1.26 microseconds. In fact, 29 June 2022 was the shortest day ever directly recorded.
But something strange appears to be occurring in the short-term trends. Since 2020, the average day has been getting longer – in other words, Earth is slowing down. This goes against a previous pattern of the average day shortening for the half-century before that.
So what’s going on? Prof Xiaodong Song and Yi Yang from Peking University in China believe they may have the answer and they think it’s Earth’s inner core.
The core spins differently from the mantle
The solid inner core sits inside the cocoon of the liquid outer core and so it’s not rigidly held in place. It’s free to spin at a different rate from the mantle and crust above. The inner core used to spin faster than the rest of the planet but Yang and Song suggest that it has slowed down recently and may even be rotating slower than the layers above.
The pair looked for seismic events that happened in the same location many years apart. In particular, they studied earthquakes erupting close to the South Sandwich Islands in the Atlantic and the resulting seismic detections in Alaska. Their paper, Multidecadal variation of the Earth’s inner-core rotation, also mentions seismic waves recorded in Montana, US, from two nuclear tests conducted at Novaya Zemlya, USSR, in 1971 and 1974.

If the core hasn’t changed, then it should reflect the seismic waves in the same way and they would look almost identical at the surface. Except that’s not what Song and Yang found. The differences they saw led them to conclude that, since 2009, the inner core has been slowing.
By comparing this data to older measurements stretching back to 1964, they conclude that this behaviour is “part of an approximately seven-decade oscillation, with another turning point in the early 1970s.” Perhaps the core speeding up and slowing down in a repeating pattern?
Why is the core slowing down?
It’s a tentative finding, one that needs more supporting data, and other researchers have put forward alternative explanations. It could be that the surface of the inner core isn’t as smooth as generally believed, for example. If it’s rougher then that could change how the inner core reflects seismic waves without needing a change in speed.
If the core does turn out to be slowing down, what could be behind it? Well, the inner core isn’t completely free to move – it’s partially pinned by the gravity of the mantle.
Some geophysicists argue that this sets up a cycle in which the inner core slows down and speeds up. Perhaps we’re just observing a short part of this cycle and it’ll speed up again very soon.
With an unprecedented number of seismometers scattered across the planet – and more being added all the time – we may not have to wait too long for answers.
What’s going on with our magnetic field?
Earth’s magnetosphere keeps us safe from the radiation pouring out of the Sun. But recent research suggests the whole thing could be about to turn upside down.
Earth’s magnetic field is a behemoth. It stretches some 65,000km towards the Sun but on the night side of the planet extends beyond six million kilometres. That means that for about a week of its month-long journey around Earth, the Moon is embedded within our magnetic field.
The core is behind all this. As heat escapes from the solid inner core it surges into the molten outer core and drives convection currents. Electrically charged material is moved around, which generates a magnetic field that flows up through the crust and out into space where it meets the solar wind – the high-energy stream of particles from the Sun. It’s this interaction that pushes our magnetosphere so far out on the night side of Earth.
The magnetosphere has kept life on Earth safe for billions of years. For centuries explorers relied on compasses aligned with it for navigation, while animals follow it to find their way too. And yet, it’s not as constant as it may seem.
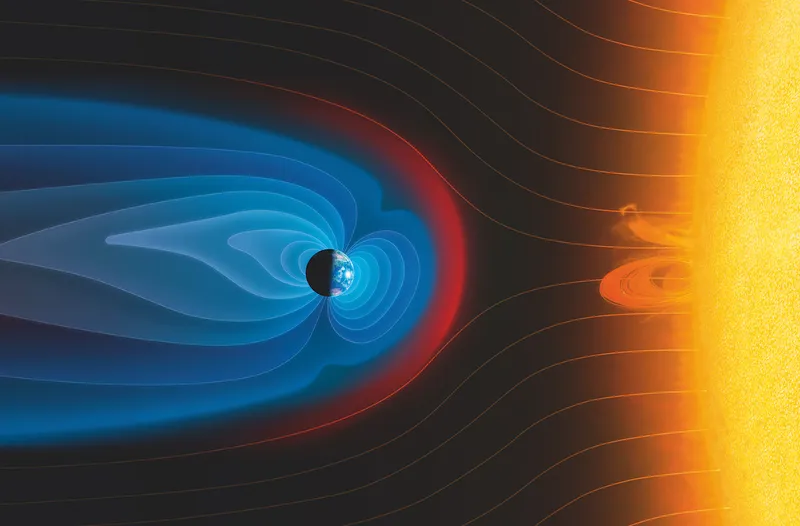
In the 1970s scientists spotted a phenomenon called geomagnetic jerks – abrupt and unpredictable changes in our magnetic field. But they only began to understand them once we started looking down at ourselves from space.
Computer simulations can help scientists
Then, in 2019, Julien Aubert of the University of Paris and Prof Christopher Finlay of the Technical University of Denmark released the results of a supercomputer simulation of the outer core. They found that waves created in the inner core spread into the outer core and cause sharp changes in the flow of liquid beneath the magnetic field. It can take 25 years for a rising blob of metal to lead to a geomagnetic jerk.
Our magnetic field can also flip. When lava cools it preserves information about the direction of the Earth’s magnetic field at the time. By analysing layers of lava, researchers have shown that, on average, the direction of our magnetic field reverses every 200,000 years.
The last flip was 780,000 years ago... and there are signs another may be on the way.
How strong is Earth's magnetic field?
According to the European Space Agency, over the last 200 years, the global average strength of our magnetic field has dropped by nine per cent. Such drops have preceded previous reversals. In some spots, the reduction has unfolded at an even more dramatic pace. Take the so-called South Atlantic Anomaly (SAA), which sits over South America. “It’s a region where geomagnetic intensity is the lowest,” according to NASA geophysicists Weijia Kuang and Terence Sabaka. But it’s also changing.
“Observations have found that the SAA is expanding and moving westward,” Kuang and Sabaka say. The field strength of the SAA also dropped by eight per cent between 1970 and 2020. What’s causing it?
“The short answer is that the SAA is due to vigorous convection in Earth’s outer core,” say Kuang and Sabaka. It’s associated with a magnetic reversal in the outer core that works against the main magnetic field.
This has some downsides. Several satellites moving through the region have failed due to the intense radiation that leaks in from space. Astronauts can’t perform spacewalks if they’re in the vicinity. The former astronaut Terry Virts even said he saw a massive flash of light, while his eyes were closed, when passing over it.
But the SAA does help geophysicists to understand what’s going on in the bowels of the planet.
According to Kuang and Sabaka, the SAA can be used to map the flow of material in the topmost part of the outer core. “The SAA forecast accuracy can [also] be used to estimate the entire core state, which is not observable from Earth’s surface or in space,” they add.
What will happen in the future?
Will it stop spinning? Will it solidify completely? And what will it mean for those of us living above it?
Earth’s core can trace its history back to the formation of the planet.
When the Sun sparked out of a cloud of interstellar gas and dust, a band of leftover material formed around it. This protoplanetary disc was laced with the iron ejected into the Universe by the cataclysmic supernovae that mark the end of the lives of the most massive stars.
Gradually, gravity fashioned this material into lumps of rock and metal called planetesimals and they smashed together to form planets. The impacts were so forceful that the rock and metal melted and gravity could round out the new object into a sphere. The heavy iron sank to the middle and the lighter rock floated to the top.
As the planet cooled, a crust formed on the surface but the iron core remained molten. This was sustained by the immense gravitational pressure of the layers above crushing down on the core.
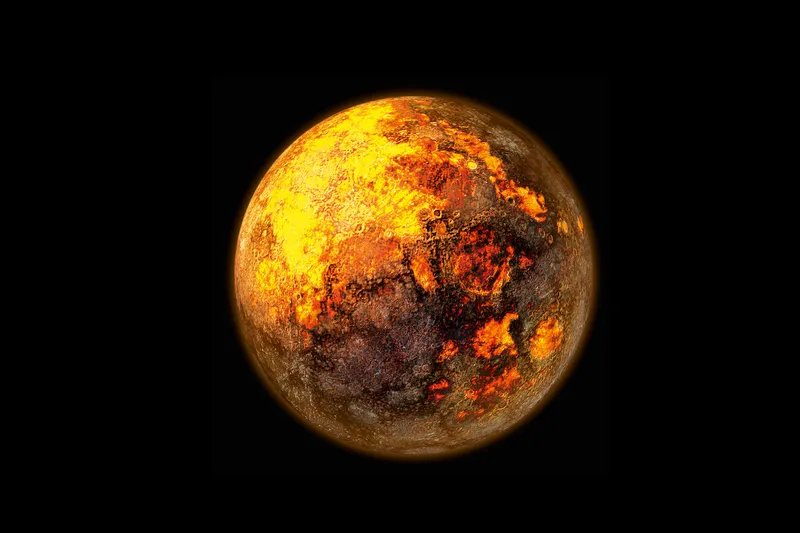
But all this happened billions of years ago and the core has been cooling ever since. “As the liquid iron in the outer core cools it slowly freezes into solid iron and becomes the inner core,” says Dr Dan Frost, a seismologist at the University of South Carolina.
How does the Earth cooling down affect the inner core?
This process adds 8,000 tonnes of iron to the inner core every second – the equivalent of the mass of the entire human population added daily. As the inner core cools, energy is transferred to the outer core, which drives convection and creates our global magnetic field.
Yet new research led by Frost is hinting that the growth of the inner core is uneven. The eastern part of the inner core lies beneath Asia and the Western Pacific, while the western part sits below the Americas and the Atlantic.
Frost’s team set about measuring the growth across these far-flung parts of the planet’s interior. It’s a tricky thing to do when all you’ve got to go on are measurements made from the surface.
“We can’t measure that the inner core is a little bit bigger today than it was yesterday, our measurements aren’t that sensitive,” Frost says. “We’re looking for evidence of movement within the inner core.”
Seismic waves can tell us what's going on
Seismic waves travelling through the inner core move faster when they move parallel to Earth’s rotation axis (roughly north-south) than when they travel parallel to the equator.
“We think this means that the crystals in the inner core are all aligned in a similar direction,” Frost says. “The way to get that alignment is if the inner core moves.” It’s similar to the way that sticks dropped into a river align with the direction of the flowing water.
Frost’s team found that the core below the Banda Sea near Indonesia is growing faster than the side beneath Brazil. The lop-sidedness doesn’t last, however. “Gravity forces [the wider part] back into the centre,” says Frost. “That flow of material would cause the crystal alignment that we see.”
Is there more to the core than meets the eye?
Frost’s work does assume that the inner core is only made up of one type of crystallised iron. We’ve already seen that other work hints at a difference between the outermost inner core and the innermost inner core.
Frost isn’t convinced of those conclusions. “There isn’t a sharp transition between an outermost and innermost inner core,” Frost says. “It’s more of a smooth transition.” Frost says that such a model is compatible with his findings.
It just goes to show that many mysteries surrounding Earth’s solid core remain unsolved. We’ve only known of its existence for less than a century. Perhaps the next century will bring more insights and a deeper understanding of the mechanism that keeps us all safe from the ravages of radiation being blasted at us from space?
Nor should we worry about the core completely solidifying any time soon. Its growth is slow. The inner core is only getting about 2mm wider every year. Although fast for a geological process, some estimates suggest it would take another 91 billion years for the molten outer core to disappear.
But don't worry; the dying Sun will fry Earth long before that happens.
Read more: